Editing our world: CRISPR and the future of genomics
The basic technology behind gene editing and a conversation between Jennifer Doudna and Siddhartha Mukherjee.
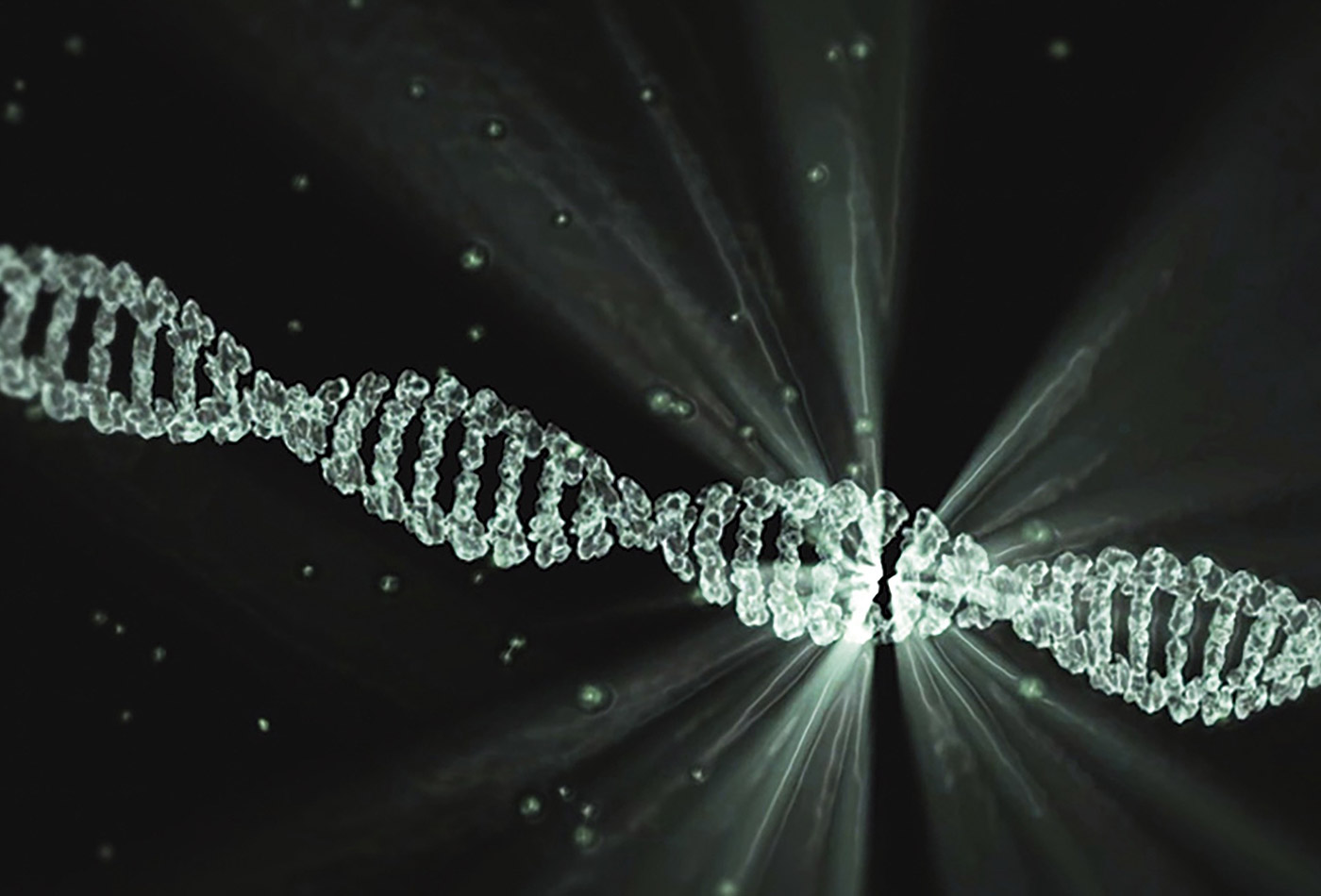
I recently attended a CRISPR talk at the University of California, Berkeley’s antique-inspired Hertz Music Hall featuring two of contemporary science’s most prominent and of-the-moment figures. Jennifer Doudna, the scientist credited with some of the greatest advancements in CRISPR technology, is a Berkeley native, at least by virtue of occupation. She is the founder of the well-known Doudna Lab as well as the director of the Innovative Genomics Institute, a joint venture between UC Berkeley and UC San Francisco. Siddhartha Mukherjee is a physician, researcher, author, and assistant professor of medicine at the University of Columbia’s Medical Center. Best known for his books, “The Emperor of All Maladies: A Biography of Cancer” and “The Gene: An Intimate History,” Mukherjee is well-versed in the intricacies of medical biology as well as the impact that advancements in genomics can have on modern medicine. This article stems from the conversation between these two influential thinkers, where the prospects for CRISPR were discussed in relation to scientific research, medical treatments, and society at large.
What Does “Gene Editing” Entail and How Is CRISPR Involved?
The term “gene editing” has been wildly popularized in the scientific community, Doudna and Mukherjee view it as the logical expression for the techniques used in the process and the genetic outcome achieved.
The general idea behind gene editing is to manipulate DNA sequences by adding, removing, or replacing sections of the genetic material. This can be achieved through the employment of various engineered nucleases, viral systems, and transposons. Engineered nucleases include Zinc Finger Nucleases (ZFNs), Transcription Activator-like Effector Nucleases (TALENs), and perhaps most notably Clustered Regularly Interspaced Short Palindromic Repeat, more commonly referred to as CRISPR. Nucleases are essentially “molecular scissors,” enzymes that segment DNA into smaller units of nucleotides through selective cleavages. When these nucleases are programmed to cut at specific DNA sites, they have the ability to bring about desired genetic modifications and therefore specific phenotypic variations.
With viral systems and transposons, genetic modification does not necessarily rely on the cut and alter technique. Instead, nucleotides are directly exchanged or transposed according to the desired end sequence. Recombinant Adeno-Associated Virus (rAAV) is a common method for altering cell lines, using the exchange of nucleotides to modify genetic sequences without causing breaks in the double-stranded DNA. Transposons likewise do not always require breaks in genetic material; they are segments of chromosomes that can be transposed into host cell DNA, either through a “cut-and-paste” or a “copy-and-paste,” to put it into processing terms.
Despite the varieties of gene editing techniques, the promising aspects of CRISPR-Cas9 in particular are its speed, economical cost, and higher accuracy. So how does CRISPR function?
To begin with, CRISPR is derived from natural processes in archaea and bacteria. Invading pathogens contain nucleic sequences that are retained in the repetitions of base sequences that make up CRISPR. These retained nucleic structures are transcribed into RNA which then instruct endonucleases to cleave the DNA of subsequent pathogens, effectively destroying harmful invaders. This concept is translated into genome editing through the protein Cas9 which utilizes a synthetic RNA guide to create targeted breakages in DNA, thereby inducing modification of the genetic material.
In the lab, CRISPR-Cas9 is transfected into a cell with a specifically designed guide RNA to facilitate the desired alterations, hence the idea of the “programmed” protein. While Cas9 unwinds the double helix, the guide RNA basepairs with one strand and simultaneously cleaves both strands of DNA.
Two labs, the Doudna Lab of UC Berkeley and the Zhang Lab of the Massachusetts Institute of Technology, have facilitated major advancements in CRISPR technology, both hoping to contribute to the contemporary biotechnology industry.
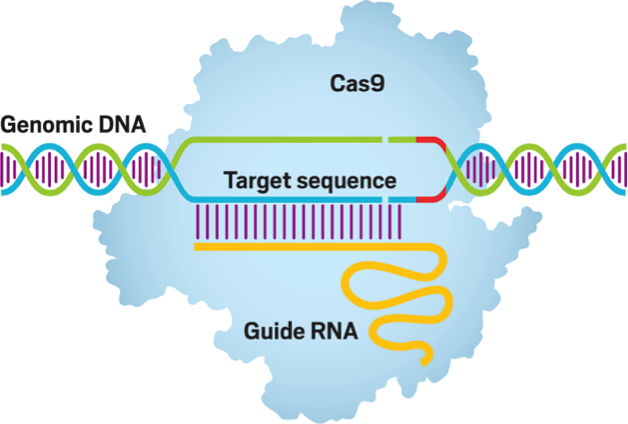
What Are Some Potential Uses of CRISPR?
The implications of CRISPR technology are far and wide. Doudna and Mukherjee have overlapping visions of its potential uses, the former seeing it as a resource for the entire biosphere and the latter viewing it as particularly effective in medical technology.
Perhaps it is Mukherjee’s clinical background that causes him to view genome editing as an invaluable tool in the medical arena. He believes that physicians are always “straddling the line between treating and curing,” but with the increasing accessibility to gene editing techniques it is possible to “think about altering DNA as a means to treat and cure.”
With a complementary perspective, Doudna sees gene editing as being “used widely across the biosphere.” She believes that with CRISPR humans possess the means to “change plants to be more nutritious or grow in environments where they otherwise wouldn’t thrive.” We can “control the spread of disease by insects” or “edit immune cells so that they are more effective at targeting cancer.” In response to sickle cell anemia, Doudna envisions “editing blood cells so that they no longer carry the disease-causing gene for sickle cell.”
These uses of CRISPR are only a small number of potential applications. Start-ups, academic research labs, at-home biohackers: CRISPR is being used in all these settings and more. Online sites sell “DIY CRISPR Kits,” YouTubers create DIY genome editing videos, CRISPR is becoming increasingly available to anyone with roughly $150 and a knowledge of the required wet lab techniques.
From a start-up perspective, there are several companies using gene editing technology to address major societal problems. Caribou Biosciences is a spin-off of the Doudna Lab, the cofounder Rachel Haurwitz being a former Ph.D. student at UC Berkeley. The company intends to use CRISPR for therapeutics, agricultural biotechnology, biological research, and industrial biotechnology. Specifically, it envisions using CRISPR in the following areas: disease models, bioproduction cell lines and fermentation strains, functional genomic screens, and plants with enhanced traits. Caribou is also partnered with Novartis and Intellia Therapeutics, paving the way for additional developments in the technology.
Nextbiotics is another company with a medical-based purpose to using CRISPR technology. Its process relies on three key points to eliminate antibiotic-resistant bacteria:
-
Sequence bacteria to identify drug resistance
-
Engineer the genome of a virus
-
Use the virus as a drug delivery system to target antibiotic resistance in bacteria
In a seemingly unconventional use of CRISPR, Stanley Qi prevented the protein from editing DNA
in order to create a platform for other molecules to affect genes by turning them on or off. Rather than changing the function of a gene, this process changes its activity. For example, the technology could stimulate heart muscles to respond effectively to a heart attack or prompt a gene to respond to abnormal cell growth.
Certainly, other applications of CRISPR will emerge; it is only a matter of deciding what is and isn’t worthwhile in using this technology.
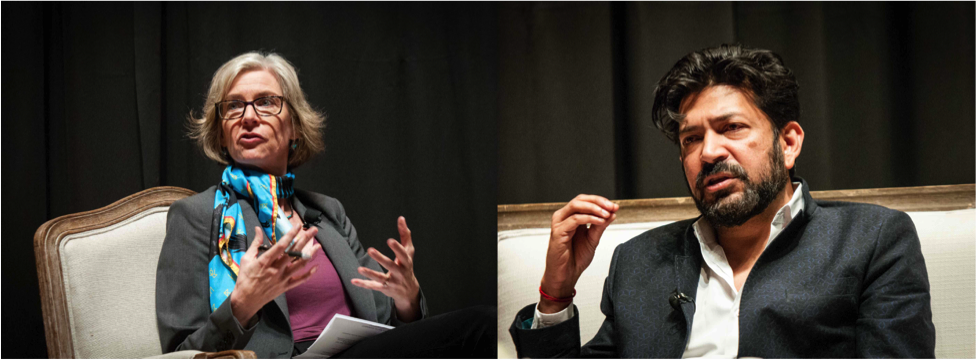
What Are the Ethical Implications Involved with CRISPR?
In 1869, almost one-hundred and fifty years ago, Frederick Miescher isolated DNA from cells for the very first time. In 1902, Archibald Garrod attributed alkaptonuria, a rare disease in which the body cannot process certain amino acids, to a recessive mutation, thereby denoting genetics as the causal factor. Not long after, in 1911, Thomas Hunt Morgan showed that chromosomes carry genes and that genetic linkage exists. All of these developments represented vast degrees of change in the information known about genetic material and have led to our greater ability to manipulate it. And with the power to manipulate comes the issue of ethics. How far can, and should, humans go in altering DNA?
Doudna and Mukherjee discuss genome alteration as a balance between “natural” and “unnatural.” From Mukherjee’s perspective, there are three major concepts we need to examine when dealing with gene editing in patient care and he summarizes these concepts with his “triangle idea.”
Firstly, Mukherjee questions if there is “extraordinary suffering involved.” This concept completely rules out the “designer baby” dilemma (i.e. using gene editing for cosmetically desired traits). Patients must have genetic conditions that produce intense distress and therefore are “ethically” targetable.
Secondly, Mukherjee desires assurance that “when we make genetic changes there is a strong degree of certainty that there is a relationship between the gene and the phenotype.” Genes are often related to many bodily functions, directly or indirectly, and unintended consequences of altering DNA is a realistic concern for scientists. Furthermore, if gene editing is performed in the germline, any modifications are passed down to future offspring.
Thirdly, Mukherjee believes that there must be a “justifiable choice.” That is, “when we intervene, the intervention bears some justification.” And this is perhaps the most important, though ambiguous, concept to evaluate because it determines the acceptable level of human influence in the genome. In an instance between treating a patient with sickle-cell anemia or giving someone the opportunity to have a child with blue versus brown eyes, there is a very clear distinction between necessity and luxury. However, not every situation is so black and white, and questionable uses of CRISPR may present themselves.
Doudna, in contrast, has had an evolved view of the ethical implications of CRISPR. “When I first started thinking about the implications of gene-editing back in the early days of this work, I felt initially very opposed to using gene-editing in embryos,” she says. “It seemed unnatural.” Doudna was particularly concerned with the idea of unintended consequences of genome altering. “A gene that we think has one certain function might only play that role in a particular context of other genes so how can we ever be sure that something we’re altering might not have unintended consequences?” she points out. However, she believes that “when there is severe suffering and we have an opportunity to change that, it may be worth doing.”
Where is CRISPR Going in the Future and How Will This Science Reach the General Public?
Undoubtedly, the point of Doudna and Mukherjee’s talk was meant to publicize CRISPR research and expand the scope of people who are familiar with gene editing. Mukherjee’s books directly connect the public to scientific information and provide in-depth educational resources in the form of easily accessible texts. It is increasingly popular to share scientific developments outside the community that these developments originate from, and Doudna and Mukherjee are leading the way.
It’s Doudna’s belief that “the concepts and ideas [associated with developments such as those regarding CRISPR] are not complicated.” She pushes for explaining scientific ideas using “language that isn’t filled with acronyms,” being that these acronyms are often a large barrier to understanding.
Doudna and Mukherjee even explore the possibility of using Hollywood to convey scientific research to a larger scale of people. Doudna questions “how do we explain what’s happening? How do we work with Hollywood?”
And though Mukherjee believes that “Hollywood gets it wrong when it tries to tell us about science,” both scientists agree that the emotional connectivity that Hollywood can establish between science and the public is valuable in itself. “Films like this make people think about science, they think about the opportunities if we develop technology to explore our solar system in ways we haven’t before,” Doudna explains.
As for the future of CRISPR, both scientists see endless possibilities.
“As of last week, in core blood stem cells we are getting gene-editing successfully in 90% of the stem cells. I suspect that the entire blood system is open for business,” says Mukherjee. He also views deep learning as an area of intersection for CRISPR work. “Deep learning is beginning to elucidate things about our genome that I did not think were possible before. Complex human phenotypes can often be explained by nudge effects.”
Doudna believes that CRISPR and gene-editing technologies “are going to help with nudge effects in the sense that they’re going to help uncover all the genes that are in those networks. There are lots of labs that are using gene-editing…to understand the genetics of disease.” Furthermore, “gene-editing will have an impact clinically in the future by being able to edit or modify multiple genes at once,” she says. Proposing the concept of a “genome surgery center,” Doudna imagines a way to “treat a large swath of people that have a particular genetic disease using one configuration of a gene-editing technology that wouldn’t require individual clinical trials for each one.”
But with all of the impressive ideas to employ CRISPR technology, there are two issues to consider. First and foremost, patenting. The conflict between the University of California, Berkeley and the Broad Institute on the matter of patenting boiled down to the following statement released by the Broad Institute:
“On February 15, 2017, the United States Patent Trial and Appeal Board (PTAB) ruled that the claims of patents granted by USPTO to Broad Institute, MIT, and Harvard concerning CRISPR editing in eukaryotic cells do not interfere with patent claims filed by UC Berkeley and the University of Vienna. UCB then appealed the PTAB’s judgment to the United States Court of Appeals for the Federal Circuit, based in Washington DC. (Broad Institute is managing the CRISPR patent portfolio on behalf of Broad, MIT, and Harvard).”
UC Berkeley’s appeal was initially discarded, but in June 2018, the Doudna-Charpentier team was awarded U.S. patent 10,000,772 for CRISPR technology with major implications in developing food security and human therapeutics.
The second concern is regarding a topic that Doudna addressed in asking “what is this [CRISPR technology] doing to help people globally versus creating even more inequalities?” Technology development has high costs and it’s difficult to incentivize pharmaceuticals to provide funding if there is no certainty of an even higher margin of profit.
As CRISPR research progresses and its applications expand, it will be intriguing to see its scope of impact as well as any further questions/concerns that may arise.
To watch the full conversation between Doudna and Mukherjee, please visit the Innovative Genomics Institute website at https://innovativegenomics.org/multimedia-library/jennifer-doudna-siddhartha-mukherjee-discuss-future-humanity/