
1.5 Units and Intensity Calculations
21
1.5 Units and Intensity Calculations
We close this chapter by first listing the important numerical
constants that are necessary for our calculations. These are
q electron charge
k Boltzmann's constant
h Planck's constant
c velocity of light
1.6 xW''^^coulombs
138xlO-^^jouleslK
6.6 X10'^^ joule-seconds
3.00 xlO^ meter I second
We also use wavelength and frequency interchangeably and note
that the wavelength X is given by c/v. Figure 1.12 encompasses the
frequencies and wavelengths that are of concern to us, namely that re-
gion from the infrared to the ultraviolet where there is usable atmo-
spheric transmission and where the photon energy hv is much greater
than the thermal energy kT at room temperature.
For convenience in calculations it is often helpful to measure these
energies in electron volts, eV, the energy gained by an electron through
a potential drop of one volt, 1.6 x
10~^^
J. In this measure there are two
simple expressions to remember. These are
10
13
30
jLim
INFRARED
VISIBLE
10
14
"H
3 jj,m
3000 nm
30,000A
ULTRAVIOLET
10
15
±-^ v(Hz)
10
16
0.3 jam
300 nm
3000A
30 nm
300A
Figure 1.12 Infrared, visible, and ultraviolet frequencies and wavelengths.
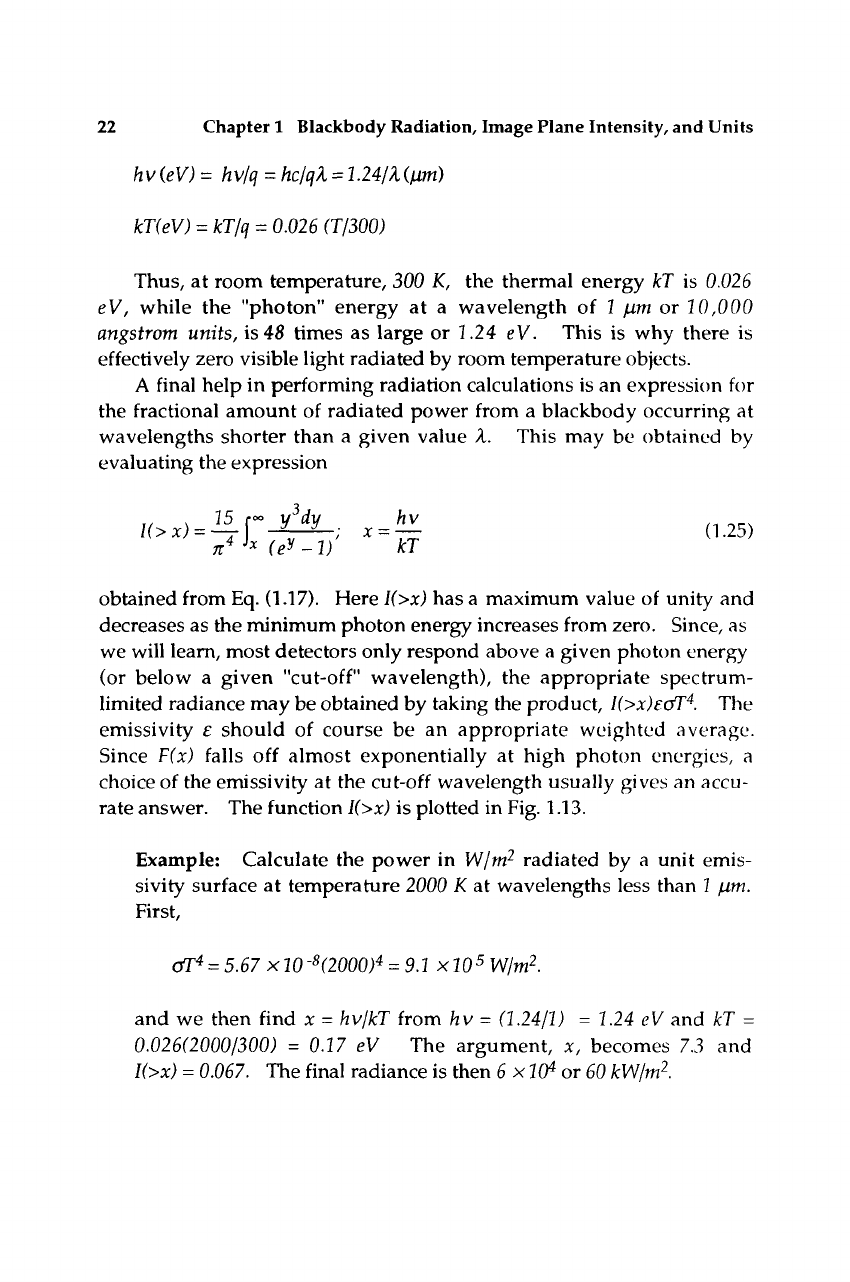
22 Chapter 1 Blackbody Radiation^ Image Plane Intensity, and Units
hvieV)= hv/q
= hc/qX =
1.24/X(jiim)
kT(eV)
= kT/q - 0.026
(T/300)
Thus,
at room temperature, 300 K, the thermal energy kT is
0.026
eV, while the "photon" energy at a wavelength of 1 jim or 10,000
angstrom units, is 48 times as large or 1.24 eV. This is why there is
effectively zero visible light radiated by room temperature objects.
A final help in performing radiation calculations is an expression for
the fractional amount of radiated power from a blackbody occurring at
wavelengths shorter than a given value X. This may be obtained by
evaluating the expression
K>,).4j-^;
,.^ 0.25)
7t^ J^ (ey - V kT
obtained from Eq. (1.17). Here
I(>x)
has a maximum value of unity and
decreases as the minimum photon energy increases from zero. Since, as
we will learn, most detectors only respond above a given photon energy
(or below a given
"cut-off"
wavelength), the appropriate spectrum-
limited radiance may be obtained by taking the product,
H>x)ecfT'^.
The
emissivity e should of course be an appropriate weighted average.
Since F(x) falls off almost exponentially at high photon energies, a
choice of the emissivity at the cut-off wavelength usually gives an accu-
rate answer. The function
l(>x)
is plotted in Fig. 1.13.
Example: Calculate the power in W/m^ radiated by a unit emis-
sivity surface at temperature 2000 K at wavelengths less than I jUm.
First,
oT^
=
5.67
xl0-^(2000)^
=
9.1
xlO^W/m^.
and we then find x = hv/kT from hv
= (1.24/1)
^ 1.24 eV and kT =
0.026(2000/300) = 0.17 eV The argument, x, becomes 7.3 and
l(>x) =
0.067.
The final radiance is then 6 xW or
60
kW/m^.
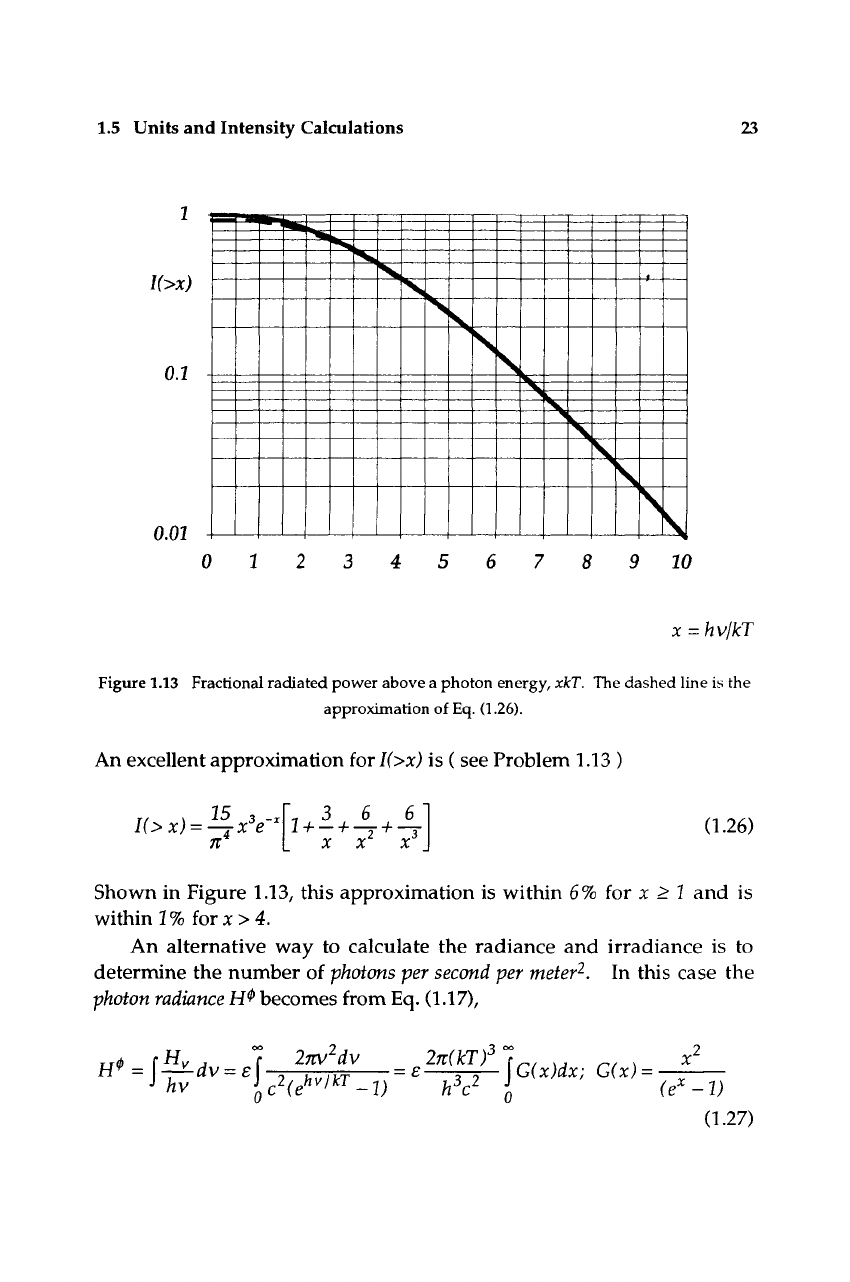
1.5 Units and Intensity Calculations 23
I(>X)
0.1
om
t-
10
X =
hv/kT
Figure 1.13 Fractional radiated power above a photon energy, xkT. The dashed line is the
approximation of
Eq.
(1.26).
An excellent approximation for
I(>x)
is ( see Problem 1.13 )
n
\ 3 6 6'
XXX
(1.26)
Shown in Figure 1.13, this approximation is within 6% for x > I and is
within
17o
for:c>4.
An alternative way to calculate the radiance and irradiance is to
determine the number of
photons
per
second
per
meter^.
In this case the
photon radiance
H^ becomes from Eq. (1.17),
litv^dv
^ = —s^dv = e ^ ,^..rj, =
e—r-^—
G(x)dx;
G(x)--
(e^'-l)
(1.27)
Get Optical Sources, Detectors, and Systems now with the O’Reilly learning platform.
O’Reilly members experience books, live events, courses curated by job role, and more from O’Reilly and nearly 200 top publishers.