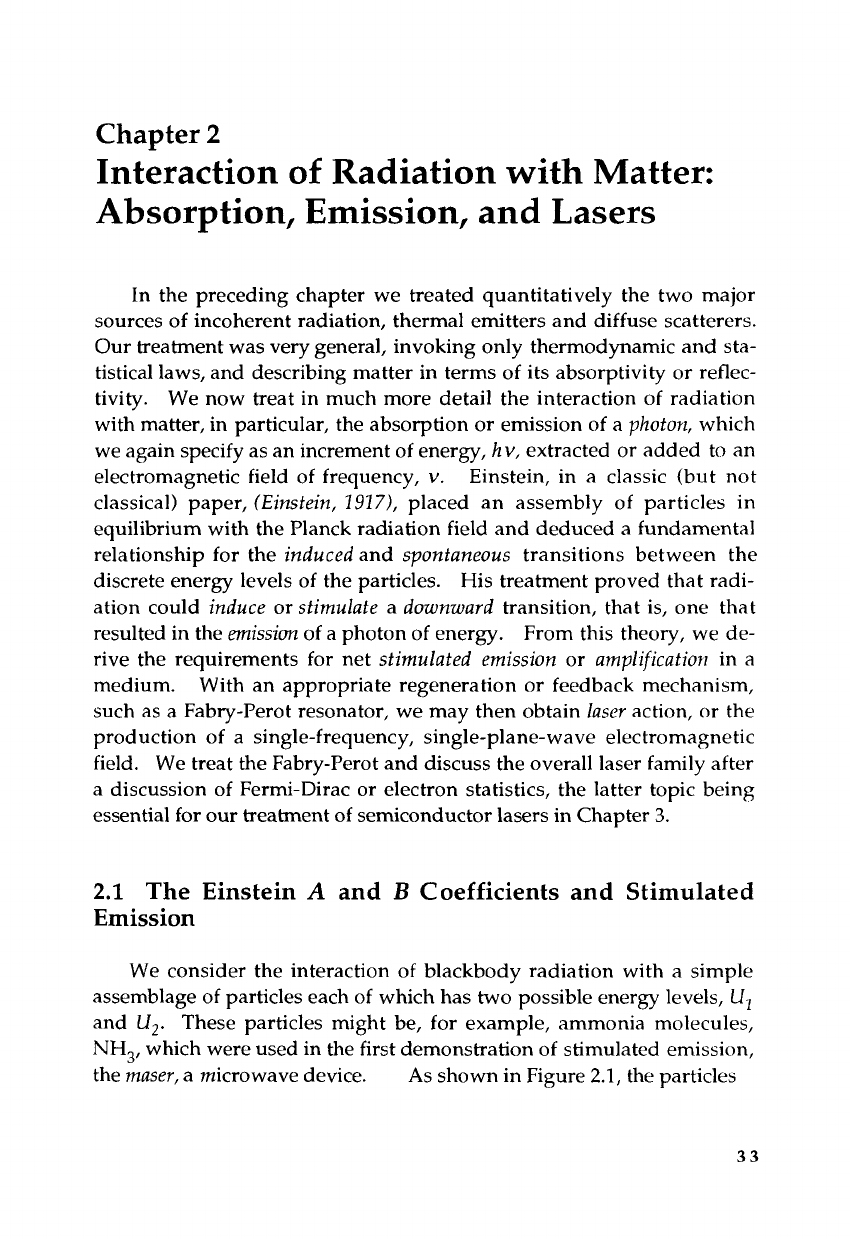
Chapter 2
Interaction of Radiation with Matter:
Absorption, Emission, and Lasers
In the preceding chapter we treated quantitatively the two major
sources of incoherent radiation, thermal emitters and diffuse scatterers.
Our treatment was very general, invoking only thermodynamic and sta-
tistical laws, and describing matter in terms of its absorptivity or reflec-
tivity. We now treat in much more detail the interaction of radiation
with matter, in particular, the absorption or emission of a photon, which
we again specify as an increment of energy, /z v, extracted or added to an
electromagnetic field of frequency, v. Einstein, in a classic (but not
classical) paper, (Einstein, 1917), placed an assembly of particles in
equilibrium with the Planck radiation field and deduced a fundamental
relationship for the induced and spontaneous transitions between the
discrete energy levels of the particles. His treatment proved that radi-
ation could induce or stimulate a downward transition, that is, one that
resulted in the
emission
of a photon of energy. From this theory, we de-
rive the requirements for net stimulated emission or amplification in a
medium. With an appropriate regeneration or feedback mechanism,
such as a Fabry-Perot resonator, we may then obtain
laser
action, or the
production of a single-frequency, single-plane-wave electromagnetic
field. We treat the Fabry-Perot and discuss the overall laser family after
a discussion of Fermi-Dirac or electron statistics, the latter topic being
essential for our treatment of semiconductor lasers in Chapter 3.
2.1 The Einstein A and B Coefficients and Stimulated
Emission
We consider the interaction of blackbody radiation with a simple
assemblage of particles each of which has two possible energy levels, L/^
and Uj' These particles might be, for example, ammonia molecules,
NH^, which were used in the first demonstration of stimulated emission,
the
maser,
a microwave device. As shown in Figure
2.1,
the particles
33

34
Chapter 2 Interaction of Radiation with Matter
U
U,
u.
N.
4
I
w
21
Ni
N
Figure 2.1 Transition rates and occupancy for states in equilibrium with blackbody field.
are distributed such that Nj are in the upper state and N^ in the lower
state.
The particles are contained in a blackbody chamber at temper-
ature T, and the occupancy probability is given by Eq. (1.1), the Boltz-
mann relation. Thus the ratio N2/N2 becomes
e'^^f^'^,
since energy
absorbing or emitting transitions between the two levels occur with a
change in electromagnetic field energy of (L/2 -
U-^)
=
hv. Defining the
transition
probabilities
per unit time as ]N^2^^^ ^2V ^^ "^'^Y write that
N^]Ni2
-
N2VJ21
since the total rates must be equal in thermal equi-
librium.
Now Einstein hypothesized that there were both spontaneous and
induced
or
stimulated
transitions. The spontaneous transitions occurred
from the upper to the lower state with a probability per unit time of A,
characteristic of the particle. In contrast the induced transition prob-
ability was assumed to be proportional to the electromagnetic spectral
energy density, u^, of Eq. (1.12), and transitions were induced in both
directions. It was this latter conjecture that was most surprising since
absorption had always been treated as the single process of excitation
from the lower to the higher energy state. As we will see, it is essential to
include the induced
downward
transitions to satisfy the rate equations,
which we write as
N2W22 = N2B22WV = N2W21 =N2(A + B2iUy) (2.1)
Get Optical Sources, Detectors, and Systems now with the O’Reilly learning platform.
O’Reilly members experience books, live events, courses curated by job role, and more from O’Reilly and nearly 200 top publishers.