Chapter 4. Time and Temperature: Cooking’s Primary Variables
EVER SINCE CAVEMEN FIRST SET UP CAMPFIRES AND STARTED ROASTING THEIR KILL, MANKIND HAS ENJOYED A WHOLE NEW SET OF FLAVORS IN FOOD. Cooking is the application of heat to ingredients to transform them via chemical and physical reactions that improve flavor, reduce chances of foodborne illness, and increase nutritional value.
From a culinary perspective, the more interesting and enjoyable changes are brought about when compounds in food undergo the following chemical reactions:
- Protein denaturation
The native form of a protein is the three-dimensional shape (conformation) assumed by the protein that is required for normal functioning. If this structure is disrupted (typically by heat or acid), the protein is said to be denatured. Changes in the shapes of proteins also alter their taste and texture.
Different proteins denature at different temperatures; most proteins in food denature in the range of 120–160°F / 49–71°C. Egg whites, for example, begin to denature at 141°F / 61°C and turn white because the shape of the denatured protein is no longer transparent to visible light. In meat, the protein myosin begins to denature around 122°F / 50°C; another protein, actin, begins to denature around 150°F / 65.5°C. Most people prefer meat cooked such that myosin is denatured while keeping the actin native.
- Maillard reaction
A Maillard reaction is a browning reaction that gives foods an aromatic and mouth-watering aroma. Usually triggered by heat, this occurs when an amino acid and certain types of sugars break down and then recombine into hundreds of different types of compounds. The exact byproducts and resulting smells depend upon the amino acids present in the food being cooked, but as an example, imagine the rich smell of the crispy skin on a roasted chicken.
For culinary purposes, the reaction generally becomes noticeable around 310°F / 154°C, although the reaction rate depends on pH, chemical reagents in the food, and amount of time at any given temperature. Many meats are roasted at or above 325°F / 160°C—at temperatures lower than this, the Maillard reaction hardly occurs.
- Caramelization
Caramelization is the result of the breakdown of sugars, which, like the Maillard reaction, generates hundreds of compounds that smell delicious. Pure sucrose (the type of sugar in granulated sugar) caramelizes at between 320–400°F / 160–204°C, with only the middle range of 356–370°F / 180–188°C generating rich flavors.
In baking, those goods that are baked at 375°F / 190° C generally have a noticeably browned exterior, while those baked at or below 350°F / 175°C remain lighter-colored.
“Great,” you’re probably thinking, “but how does knowing any of this actually help me cook?”
You can tell when something is done cooking by understanding what reactions you want to trigger and then detecting when those reactions have occurred. Cooking a steak? Check the internal temperature with a thermometer; once it’s reached 140°F / 60°C, the myosin proteins will have begun to denature. Baking crispy chocolate chip cookies at 375°F / 190°C? Open your eyes and keep your nose online; the cookies will be just about done when they begin to turn brown and you’re able to smell the caramelization occurring. Really, it’s that simple. Foods are “done” when they achieve a certain state, once they have undergone the desired chemical reactions. As soon as the reactions have occurred, pop the food out; it’s done cooking.
Note
A small but critical detail: as we’ll discuss elsewhere, proteins don’t simultaneously denature at a given temperature. Denaturation is a function of duration of exposure at a given temperature. And there are many different types of proteins in different foods, each with its own temperature/time response rate.
Smell, touch, sight, sound, taste: learn to use all of your senses in cooking. Meat that has been cooked until it is medium rare—a point at which myosin has denatured and actin has yet to denature—will feel firmer and also visibly shrink. The bubbling sound of a sauce that’s being boiled and reduced will sound different once the water is mostly evaporated, as bubbles pushing up through the thicker liquid will have a different sound. Bread crust that has reached the temperatures at which Maillard reactions and caramelization occur will smell wonderful, and you’ll see the color shift toward golden brown. By extension, this also means that the crust of the bread must reach a temperature of 310°F / 155°C before it begins to turn brown, which you can verify using an IR thermometer. (Bread flour has both proteins and sugars, so both caramelization and Maillard reactions occur during baking.)
This chapter shows you when and how these changes occur so that you can become comfortable saying, “It’s done!” We’ll start by looking at the differences between the common sources of heat in cooking and how differences in the type of heat and temperatures impact cooking. Since one of the main reasons for cooking is reducing the chances of foodborne illness, we’ll also discuss the key issues in food safety, including a look at how to manage bacterial contamination and parasites, along with some example recipes to demonstrate the principles behind food safety. The remainder of the chapter will then examine a number of key temperature points, starting with the coldest and ending with the hottest, discussing the importance of each temperature point and giving example recipes to illustrate the reactions that occur at each of these temperatures.
As with most recipes in this book, the recipes here are components, not necessarily entire dishes or meals unto themselves. Create your own combinations as you like! It’s usually easier to take each of the components in a dish and cook them separately: veggies in one pan, meats or proteins in another, and starches in a third. This allows you to isolate the variables for each component, then combine them at the end. Eggplant Parmesan might be your favorite dish, but if you’re new to cooking, it’s probably not the best place to start to learn about the reactions taking place.
Finally, cooking and baking share an axiom with coding and product development: it’s done when it’s done—not when the timer goes off. One of the best tips I can offer for improving your skills in the kitchen is to “calibrate” yourself: take a guess if something is done and then check, taking note of what your senses, especially smell and sight, notice in the process.
Note
Timers are great guides for reminding you to check on a dish and a good safety net in case you’re like me and absentmindedly wander off occasionally. But timers are only a proxy for monitoring the underlying reactions. Given a fillet of fish that is done when its core temperature reaches 140°F / 60°C—which might take about 10 minutes—the primary variable is temperature, not time. If the fish is slow to heat up, regardless of the timer going off at the 10-minute mark, it won’t be done yet. Not to knock timers entirely: they’re a great tool, especially in baking, where the variables are much more controlled and thus the time needed to cook can be more accurately prescribed. But don’t be a slave to the timer.
Cooked = Time * Temperature
Since the primary chemical reactions in cooking are triggered by heat, let’s take a look at a chart of the temperatures at which the reactions we’ve just described begin to occur, along with the temperatures that we commonly use for applying heat to food:
There are a few “big picture” things to notice about these common temperatures in cooking. For one, notice that browning reactions (Maillard reactions and caramelization) occur well above the boiling point of water. If you’re cooking something by boiling it in a pot of water or stewing it in liquid, it’s impossible for high-heat reactions to occur, because the temperature can’t go much above 216°F / 102°C, the boiling point of moderately salted water. If you’re cooking a stew, such as the simple beef stew recipe in Chapter 2 (Simple Beef Stew), sear the meats and caramelize the onions separately before adding them to the stew. This way, you’ll get the rich, complex flavors generated by these browning reactions into the dish. If you were to stew just the uncooked items, you’d never get these high-heat reactions.
Another neat thing to notice in the temperature graph is the fact that proteins denature in relatively narrow temperature ranges. When we cook, we’re adding heat to the food specifically to trigger these chemical and physical reactions. It’s not so much about the temperature of the oven, grill, or whatever environment you’re cooking in, but the temperature of the item of food itself.
Which brings us to our first major aha! moment: the most important variable in cooking is the temperature of the food itself, not the temperature of the environment in which it’s being cooked. When grilling a steak, the temperature of the grill will determine how long it takes the steak to come up to temperature, but at the end of the day, what you really want to control is the final temperature of the steak, to trigger the needed chemical reactions. For that steak to be cooked to at least medium rare, you need to heat the meat such that the meat itself is at a temperature of around 135°F / 57°C.
Heat Transfer and Doneness
The idea that you can just cook a steak any old way until it reaches 135°F / 57°C sounds too easy, so surely there must be a catch. There are a few.
For one, how you get the heat into a piece of food matters. A lot. Clearly the center of the steak will hit 135°F / 57°C faster when placed on a 650°F / 343°C grill than in a 375°F / 190°C oven. The hotter the environment, the faster the mass will heat up, thus the rule of thumb: “cooking = time * temperature.” Consider the internal temperatures of steak cooked two ways, grilled and oven-roasted:
Cooking a steak on a grill takes less time than in an oven, because energy is transferred faster in the hotter environment of the grill. Note that the error tolerance of when to pull the meat off the grill is smaller than pulling the meat from the oven, because the slope of the curve is steeper. That is, if t1 is the ideal time at which to pull the steak, leaving it for t1+2 minutes will allow the temperature of the grilled steak to overshoot much more than one cooked in the oven.
This is an oversimplification, of course: the graph shows only the temperature at the center of the mass, leaving out the “slight” detail of the temperature of the rest of the meat. (It also doesn’t consider things like rate of heat transfer inside the food, water in the meat boiling off, or points where proteins in the meat undergo phase changes and absorb energy without a change in temperature.)
Another thing to realize about heat transfer is that it’s not linear. Cooking at a higher temperature is not like stepping on the pedal to get to the office faster, where going twice as fast will get you there in half the time. Sure, a hotter cooking environment like a grill will heat up the outer portions of the steak faster than a relatively cooler environment like an oven. But the hotter environment will continue to heat the outer portions of the steak before the center is done, resulting in an overcooked outer portion compared to the same size steak cooked in an oven to the same level of internal doneness.
What’s the appeal of cooking on a hot grill, then? For the right cut of meat, you can keep a larger portion of the center below the point at which proteins become tough and dry (around 170°F / 77°C) while getting the outer portion up above 310°F / 154°C, allowing for large amounts of Maillard reactions to occur. That is, the grill helps give the outside of the steak a nice brown color and all the wonderful smells that are the hallmark of grilling—aromas that are the result of Maillard reactions. The outside portion of grilled meat will also have more byproducts from the Maillard reactions, resulting in a richer flavor.
Juggling time and temperature is a balancing act between achieving some reactions in some portions of the meat and other reactions in other parts of the meat. If you’re like me, your ideal piece of red meat is cooked so that the outer crust is over 310°F / 155°C and the rest of the meat is just over 135°F / 57°C, with as little of the meat between the crust and the center as possible being above 135°F / 57°C. The modern technique of sous vide cooking can be used to achieve this effect; we’ll cover this in Chapter 7.
Temperature gradients
This balancing act—getting the center cooked while not overcooking the outside—has to do with the rate at which heat energy is transferred to the core of a food. Since cooking applies heat to foods from the outside in, the outer portions will warm up faster, and because we want to make sure the entire food is at least above a minimum temperature, the outside will technically be overcooked by the time the center gets there. This difference in temperature from the center to outer edges of the food is referred to as a temperature gradient.
Note
Choose the method of cooking to match the properties of the food you are cooking. Smaller items—skirt steak, fish fillets, hamburgers—work well at high heats. Larger items—roasts, whole birds, meatloaf—do better at moderate temperatures.
All parts of our example steak are not going to to reach temperature simultaneously. Because grill environments are hotter than ovens, the temperature delta between the environment and the food is larger, so foods cooked on the grill will heat up more quickly and have a steeper temperature gradient.
Carryover
Carryover in cooking refers to the phenomenon of continued cooking once the food is removed from the source of heat. While this seems to violate a whole bunch of laws of thermodynamics, it’s actually straightforward: the outer portion of the just-cooked food is hotter than the center portion, so the outer portion will transfer some of its heat into the center. You can think of it like pouring hot fudge sauce on top of ice cream: even though there’s no external heat being added to the system, the ice cream melts because the hot fudge raises its temperature.
The amount of carryover depends upon the mass of the food and the heat gradient, but as a general rule, I find carryover for small grilled items is often about 5°F / 3°C. When grilling a steak or other “whole muscle” meat, pull it when it registers a few degrees lower at its core than your target temperature and let it rest for a few minutes for the heat to equalize.
Note
To see how this works, try using a kitchen probe thermometer to record the temperature of a steak after removing it from the grill once it reaches 140°F / 60°C, recording data at 30-second intervals. You should see the core temperature peak at around 145°F / 63°C three minutes into the rest period for a small steak.
Methods of Heat Transfer
There are three methods of transferring heat into foods: conduction, convection, and radiation. While the heating method doesn’t change the temperature at which chemical reactions occur, the rate of heat transfer is different among them, meaning that the length of time needed to cook identical steaks via each method will be different. The table below shows the common cooking techniques broken out by their primary means of heat transfer.
Conduction
Conduction is the simplest type of heat transfer to understand because it’s the most common: it’s what you experience whenever you touch a cold countertop or grasp a warm cup of coffee. In cooking, those methods that transfer heat by direct contact between food and a hot material, such as the hot metal of a skillet, are conduction methods. Dropping a steak onto a hot cast iron pan, for example, causes thermal energy from the skillet to be transferred to the colder steak as the neighboring molecules distribute kinetic energy in an effort to equalize the difference in temperature. For more on thermal conductivity, see the Metals, Pans, and Hot Spots in Chapter 2.
Conduction | Convection | Radiation | |
Description | Heat passes by direct contact between two materials | Heat passes via movement of a heated material against a colder material | Heat is transferred via electromagnetic radiation |
Example | Steak touching pan; pan touching electric burner | Hot water, hot air, or oil moving along outside of food | Infrared radiation from charcoal |
Uses | Sautéing Searing | Dry heat methods: - Baking/roasting - Deep-fat frying Wet heat methods: - Boiling - Braising/water bath - Pressure cooking - Simmering/poaching - Steaming | Microwaving Broiling Grilling |
Convection
Convection methods of heat transfer—baking, roasting, boiling, steaming—all work by circulating a hot material against a cold one, causing the two materials to undergo conduction to transfer heat. In baking and roasting, the hot air of the oven imparts the heat; in boiling and steaming, it’s the water that does this.
Those heat methods that involve water are called wet heat methods; all others fall into the dry heat category. One major difference between these two categories is that wet methods don’t reach the temperatures necessary for Maillard reactions or caramelization (with the exception of pressure cooking, which does get up to temperature while remaining moist). The flavorful compounds produced by Maillard reactions in grilled or oven-roasted items won’t be present in braised or stewed foods: steamed carrots, for example, won’t undergo any caramelization, leaving the food with a subtler flavor. Brussels sprouts are commonly boiled and widely hated. Next time you cook them, quarter them, coat with olive oil and sprinkle with salt, and cook them under a broiler set to medium.
Note
Water is an essential material in cooking, and not just for its heat transfer properties. Rice cookers work by noticing when the temperature rises above 212°F / 100°C. At that point, there’s no water left, so they shut off.
Another key difference between most of the dry versus wet methods is the higher speed of heat transfer typical in wet methods. Water conducts heat roughly 23 times faster than air (air’s coefficient of thermal conductivity is 0.026, olive oil’s is 0.17, and water’s is 0.61), which is why hard-cooked eggs finish faster in a wet environment even at a lower temperature.
Note
Try it! Cook one egg for 30 minutes in a 325°F / 165°C oven and another for 10 minutes in a 212°F / 100°C water bath. You need to leave the egg in the oven for 20 minutes longer to get the same results.
One exception to this wet-is-faster-than-dry rule is deep-fat frying. Oil is technically dry (there’s no moisture present), but for culinary purposes it acts a lot like water: it has a high rate of heat transfer with the added benefit of being hot enough to trigger a large number of caramelization and Maillard reactions. (Mmm, donuts!)
Wet methods have their drawbacks (including, depending on the desired result, the lack of the aforementioned chemical reactions). While the subtler flavors achieved without browning reactions can be desirable, as in a gently cooked piece of fish, it’s also much easier to overcook foods with wet methods. When cooking meat, the hot liquid interacting with it can quickly raise its temperature above 160°F / 71°C, the point at which a significant percentage of the actin proteins in meats are denatured, giving the meat a tough, dry texture. For pieces of meat with large amounts of fat and collagen (such as ribs, shanks, or poultry legs), this isn’t as much of an issue, because the fats and collagen (which converts to gelatin) will mask the toughness brought about by the denatured actin. But for leaner cuts of meat, especially fish and poultry, take care that the meat doesn’t get too hot! The trick for these low-collagen types of meats is to keep your liquids at a gentle simmer, around 160°F / 71°C, and minimize the time that the meat spends in the liquid.
Note
Even water in its gaseous form—steam—can pack a real thermal punch. While it doesn’t conduct heat anywhere nearly as quickly as water in its liquid form, steam gives off a large amount of heat due to the phase transition from gas to liquid, something that air at the same temperature doesn’t do. As the steam comes into contact with colder food, it condenses, giving off 540 calories (not to be confused with “food calories,” which are technically kilocalories) of energy per gram of water, causing the food to heat up that much more quickly (1 calorie raises the temperature of 1 gram of water by 1°C).
Steamed vegetables, for example, cook quickly not just because they’re in a 212°F / 100°C environment, but also because the water vapor condensing on the food’s surface imparts a lot of energy. Cheetos, like most “extruded brittle foams” we eat, gain their puff by being expelled under pressure and heat, which causes them to “steam puff.” (Think of it as the industrial version of popping popcorn.) There’s a lot of energy in steam. For this reason, when pouring boiling water through a colander over a sink, you should be sure to pour away from yourself so that the steam cloud (and any splashed liquid) doesn’t condense on your face!
Radiation
Radiant methods of heat transfer impart energy in the form of electromagnetic energy, typically microwaves or infrared radiation. The warmth you feel when sunlight hits your skin is radiant heat.
In cooking, radiant heat methods are the only ones in which the energy being applied to the food can be either reflected or absorbed by the food. You can use this reflective property to redirect energy away from parts of something you’re cooking. One technique for baking pie shells, for example, includes putting foil around the edge, to prevent overcooking the outer ring of crust. Likewise, if you’re broiling something, such as a chicken, and part of the meat is starting to burn, you can put a small piece of aluminum foil directly on top of that part of meat. It might be a hack, but in a pinch it’s a decent way to avoid burning part of a dish, and nobody but you, me, and everyone else who reads this book will ever know.
Combinations of heat
The various techniques for applying heat to food differ in other ways than just the mechanisms of heat transfer. In roasting and baking we apply heat from all directions, while in searing and sautéing heat is applied from only one side. This is why we flip pancakes (stovetop, heat from below) but not cakes (oven, heat from all directions). The same food can turn out vastly different under different heat conditions. Batter for pancakes (conduction via stovetop) is similar to that for muffins (convection via baking) and waffles (conduction), but the end result differs widely.
To further complicate things, most cooking methods are actually combinations of different types of heat transfer. Broiling, for example, primarily heats the food via thermal radiation, but the surrounding air in the oven also heats up as it comes in contact with the oven walls, then comes in contact with the food and supplies additional heat via convection. Likewise, baking is primarily convection (via hot air) but also some amount of radiation (from the hot oven walls). “Convection ovens” are nothing more than normal ovens with a blower inside to help move the air around more quickly. All ovens are, by definition, convection ovens, in the sense that heat is transferred by the movement of hot air. Adding a fan just moves the air more quickly, leading to a higher temperature difference at the surface of the (cold) food you’re cooking.
To a kitchen newbie, working with combinations of heat might be frustrating, but as you get experience with different heat sources and come to understand how they differ, you’ll be able to switch methods in the middle of cooking to adjust how a food item is heating up. For example, if you like your lasagna like I do—toasty warm in the middle and with a delicious browned top—the middle needs to get hot enough to melt the cheese and allow the flavors to meld, while the top needs to be hot enough to brown. Baking alone won’t generate much of a toasted top, and broiling won’t produce a warm center. However, baking until it’s almost done and then switching to the broiler achieves both results.
Note
The convenience food industry cooks with combinations of heat, too, cooking some foods in a hot oven while simultaneously hitting them with microwaves and infrared radiation to cook them quickly.
When cooking, if something isn’t coming out as you expect—too hot in one part, too cold in another—check to see whether switching to a different cooking technique can get you the results you want.
If you’re an experienced cook, try changing heat sources as a way of creating a challenge for yourself: adapt a recipe to use a different source of heat. In some cases, the adaptation is already common—pancake batter, when deep-fat fried, is a lot like funnel cakes. But try pushing things further. Eggs cooked on top of rice in a rice cooker? Chocolate cookies cooked in a waffle iron? Fish cooked in a dishwasher? (See Cooking in the...Dishwasher?.) Why not?
It might be unconventional, but heat is heat. Sure, different sources of heat transfer energy at different rates, and some are better suited to transitioning the starting thermal gradient (edge to center) of the food to the target thermal gradient. But there are invariably similar enough heat sources worth trying. And you can push it pretty far: fry an egg on your CPU, or cook your beans and sausage on an engine block like some long-haul truck drivers do! As a way of getting unstuck—or just playing around—it’s fun to try.
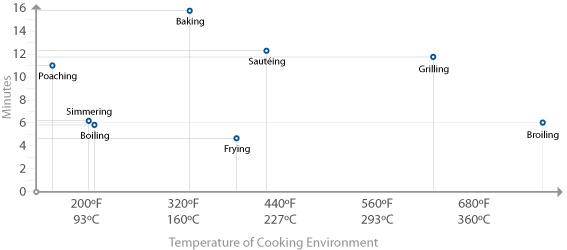
Cooking methods plotted by rate of heat transfer. This plot shows the amount of time it took to heat the center of uniformly sized pieces of tofu from 40°F / 4°C to 140°F / 60°C for each cooking method. Pan material (cast iron, stainless steel, aluminum) and baking pan material (glass, ceramic) had only minor impact on total time for this experiment and are not individually listed.
Foodborne Illness and Staying Safe[3]
The American food supply is one of the most interconnected and interdependent ones in the world. As I write this, I’m eating my morning bowl of cereal, yogurt, bananas, and almonds. The muesli cereal is from Switzerland, the yogurt local to New England, the bananas from Costa Rica, and the almonds from California. The only direction from which food hasn’t come 3,000 miles is north, and that’s probably only because not much grows at the North Pole!
As our food system has become more interconnected, the number of people that can be affected by a mistake in handling food has also increased. Today, a single bad batch of water sprayed onto a field of spinach can sicken hundreds of American consumers because that crop can be transported thousands of miles and make its way into so many dishes before the contamination is noticed.
Handling food carefully—taking note of what has been washed in the case of produce and cooked in the case of meats, and being careful to avoid cross-contamination—is among the easier ways of keeping yourself healthy.
Bacteria related to common foodborne illnesses begin to multiply above 40°F / 4.4°C. The standard food safety rule provided by the FDA for mitigating foodborne illnesses from bacteria states that food should not be held between the temperatures of 40°F / 4.4°C and 140°F / 60°C for more than two hours. Below 40°F / 4.4°C, the bacteria remain viable but won’t have a chance to multiply to a sufficient quantity to bother us. Above 140°F / 60°C, the bacteria won’t be able to survive long. (Bacterial spores, however, can.)
This is called the “danger zone rule,” and as you’d probably imagine, a vast simplification of what’s really going on in the bacterial world. Still, as an easy safety rule there’s really no reason to violate it, because there are few dishes that I can think of that actually need to violate it to be made.
Note
For those recipes that say to marinate meat at room temperature: don’t! Let it marinate in the fridge.
Keep in mind that it’s cumulative time here that matters. Say you buy a chicken at the store, and that it was kept chilled the entire time before you picked it up. Between the time you put it in the cart and when you stick it in your fridge, it’ll be in a warmer environment, and any time it spends above the temperature at which bacteria begin to multiply will increase the bacterial count in the meat.
While cooking food kills off most of the bacteria, a minor (yet safe) number can survive even post-cooking. Given the right temperature range, they can reproduce back up into unsafe quantities. When cooking, stick any leftovers in the fridge right away, as opposed to letting them sit around until post-meal cleanup. The bacterial level is all about exposure—the amount of time and rate of multiplication at a given temperature.
Note
This is why you should defrost large pieces of meat in the fridge overnight. Letting it thaw, even under cold running water, can take too long to be safe—unless your cold water happens to be below 40°F / 4.4°C!
One detail this rule glosses over is that some bacteria can reproduce at lower temperatures. Luckily, most bacteria related to foodborne illness don’t multiply very quickly at near-freezing temperatures, but other types of bacteria do. Spoilage-related bacteria, for example, are happy breeding down to freezing temperatures. These are the ones that cause milk to go bad even below 40°F / 4.4°C and break down the flesh in things like raw chicken, causing uncooked meats to go bad after a few days. The danger zone rule addresses only the common pathological bacteria, which don’t reproduce very quickly at the temperature of your fridge.
Another area that the danger zone rule glosses over is the different rates of reproduction at different temperatures. Salmonella, for example, is happiest breeding around 100°F / 37.8°C. It’s not like the bacteria go from zero multiplication at 40°F / 4.4°C to full-on party mode at 41°F / 5°C; it’s a gradual ramp up to an ideal breeding temperature. The two-hour window is given for the worst-case scenario: that the food is being held at the ideal breeding temperature for the most aggressive of the common bacteria, Bacillus cereus.
Since food safety codes are currently adopted on a state-by-state level, some states still use a danger zone rule of “40 to 140 for four hours,” on the basis that B. cereus accounts for only a minor amount of foodborne illness and that a four-hour exposure isn’t likely to produce much risk. If you’re getting the impression that food safety is a probability game, you’re right. The rules reduce the odds to an acceptable level. Still, that lunch you took to work and forgot to toss in the fridge is probably safe, given that the total amount of bacterial multiplication is likely to be well below the level necessary to trigger any sort of foodborne illness.

The danger zone rule suggests that bacteria multiply right up until 140°F / 60°C, whereas the real multiplication rates of foodborne illness-related bacteria follow a curve with an ideal breeding range in the middle. Of the foodborne pathogens listed in the FDA’s “Bad Bug Book,” Bacillus cereus has the highest survival temperature, at 131°F / 55°C.
“But wait,” you might be saying. “What about all that food in the pantry? Why doesn’t it go ‘bad’?” There are other factors that bacteria need in order to multiply. The acronym FAT TOM is commonly used to describe the six factors necessary for multiplication:
- F = Food
Bacteria need proteins and carbohydrates to multiply. No food, no multiplication.
- A = Acidity
Bacteria can only survive in certain pH ranges. Too acidic or basic, and the proteins in the bacteria denature.
- T = Temperature
Too cold, and the bacteria effectively sleep. Too hot, and they die.
- T = Time
Bacteria have to have enough time to multiply to a sufficient quantity to overwhelm our bodies.
- O = Oxygen
As with pH levels, bacteria will reproduce only if sufficient oxygen is present, or, for anaerobic bacteria (e.g., Clostridium botulinum), if no oxygen is present. Keep in mind that vacuum-packed bags are not necessarily devoid of oxygen.
- M = Moisture
Bacteria need water to reproduce. Food scientists use a scale called water activity, which is a measure of the freely available water in a material (from 0 to 1). Bacteria need a water activity value of 0.85 or greater to multiply.
The reason so many pantry goods are “shelf stable” is either low moisture content (crackers, dry goods like beans and grains, oils, even jams and jellies in which the sugar is hygroscopic and “holds on to” the water) or acidity (pickled items, vinegars). Given these six variables, you can see why some foods don’t need refrigeration. When in doubt, though, stick it in the fridge, which you should keep on the chilly side (34–36°F / 1–2°C).
Note
Keep basic physics in mind. Placing a large pot of hot soup into the fridge will warm up all the contents of the fridge until the evaporator has a chance to transfer the heat back out. Refrigerators are made to keep things cool, not to chill things, so when you’re storing a large quantity of hot food, place it in an ice bath first to chill it and then transfer it to the fridge once it’s cooled down.
There’s one variable that deserves special mention, because of the potentially fatal consequences, and that’s the oxygen level needed for bacterial growth. Specifically, anaerobic environments—ones without oxygen—are necessary for some types of bacteria to multiply. Oil creates an anaerobic environment, but by itself doesn’t provide any moisture for bacteria to grow. But with the addition of something like a raw clove of garlic, an anaerobic environment is created, and the garlic provides the food and moisture necessary for anaerobic bacteria to thrive when given sufficient time at the right temperatures.
A garlic clove stored in oil can become the perfect breeding ground for botulism, the illness caused by Clostridium botulinum. And since C. botulinum doesn’t produce any noticeable odor, there’s little to tip you off that the food is teeming with bacteria and their toxins. The toxins generated by C. botulinum are much more heat stable than the bacteria themselves and might remain active through the cooking process. And now to scare you senseless, the botulinum toxin is the most acutely toxic substance known: a dose as small as ~250 nanograms—1/120,000th the weight of a grain of rice—will do you in.
If you’re going to make something like duck confit or homemade jam the traditional way—cooking it and then sealing it for storage at room temperature—make darn sure you get the food hot enough to sterilize it and that you avoid recontaminating it after it’s cooked and before it’s sealed. To be safe, stick it in the fridge and treat it as perishable. Note that sterilization means completely eradicating any bacteria, as opposed to pasteurization, which reduces bacteria to “safe” levels for near-term consumption. Sterilized milk can be left at room temperature indefinitely; pasteurized milk cannot.
There’s one last aspect I should mention in this brief primer on food safety, and that’s the risk/consequences equation. While following food safety rules reduces the risk of illness, it doesn’t completely eliminate risk. For most of us, the consequence of contracting most foodborne illnesses is gastrointestinal distress—diarrhea, vomiting, muscle spasms, and the like. However, for those who are in an at-risk group—anyone for whom having foodborne illness can lead to further complications—the consequences of a bout of foodborne illness can be much, much greater, so the acceptable risks are accordingly much lower. If you are cooking for someone who is elderly, extremely young, pregnant, or immunocompromised, be extra vigilant with regard to food safety issues and skip dishes that have higher risks (e.g., raw egg in Caesar salads, unpasteurized cheeses, suspect meats that might be past their expiration dates).
For additional information on food safety and bacteria, check out the FDA’s “Bad Bug Book,” available online at http://www.fda.gov/Food/FoodSafety/FoodborneIllness/. Also, Texas A&M University’s Department of Horticultural Sciences maintains a good overview page at http://aggie-horticulture.tamu.edu/extension/poison.html.
While bacteria are the most common and easiest-to-manage cause of foodborne illnesses, they’re not alone: viruses, molds, toxins, and contaminants are also of concern. Toxins and contaminants are primarily issues for producers of foods, so as a consumer you’re (mostly) off the hook for those. (If you grow your own veggies, test your soil for contaminants.) Besides proper cooking, the best way to combat bacteria, mold, and viruses is good washing and avoiding cross-contamination:
Wash your hands! And don’t double-dip with the same spoon when tasting a dish.
Nuke your sponges (rinse them and give them two minutes on high) or run them through the dishwasher weekly. Better yet, use towels and allow them to dry completely between uses.
When working with raw meats, be careful not to use the same towel for wiping your hands that you then use to wipe with post-cooking.
Wash can lids before opening them. Wash the can opener, too. The blade picks up food while cutting through the lid.
How to Prevent Foodborne Illness Caused by Bacteria
Salmonella: it’s the poster child of foodborne illness. And for good reason. Salmonella accounts for a full 30% of the roughly 1,800 deaths due to foodborne illnesses per year in the U.S.—more than any other cause in this category. Salmonella’s ideal breeding temperature? Around 100°F / 38°C—close to body temperature. Clearly it likes us. And according to some reports, the most likely food that’ll harbor the bacteria in our modern food supply isn’t chicken or meats, but produce. Wash your veggies!
The odds of dying from foodborne illness are actually surprisingly low, especially considering the media attention given to it. But the media attention isn’t unmerited: 1 in 8 of us will contract illnesses from foods in any given year, and about 1% of those cases will require hospitalization, according to the U.S. Centers for Disease Control and Prevention (CDC).
Contracting a foodborne illness is a game of probabilities: a single bacterium of salmonella isn’t likely to cause a problem, but given a few dozen cells, the odds change. E. coli is similar: only a few bacteria are necessary for the possibility of infection. A few strains are decidedly nasty, O157:H7 being the most talked about.
It’s not always “just a handful” of cells, though. Contracting listeriosis requires ingesting somewhere around 1,000 organisms of Listeria monocytogenes, which tends to be present in animal products and multiplies at temperatures as low as 34°F / 1°C. Luckily, listeriosis isn’t an issue for many of us, but it can cause complications for at-risk groups—especially pregnant women, where the baby is at risk. This is why pregnant women are told to avoid foods such as soft and surface-ripened cheeses, deli salads, raw milk, hot dogs, and shrimp; to ensure that chicken is thoroughly cooked; and to be careful with previously cooked ready-to-eat foods.
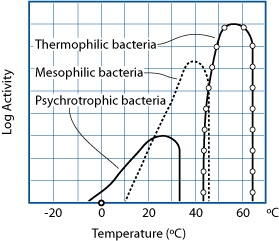
GRAPH BASED ON E. ANDERSEN, M. JUL, AND H. RIEMANN (1965), “INDUSTRIEL LEVNEDSMIDDEL-KONSERVERING,” COL. 2, KULDEKONSERVERING, COPENHAGEN: TEKNISK FORLAG)
Bacteria can be grouped into three broad categories, based on the temperature at which they are most active. There are bacteria that remain active in food above 122°F / 50°C, but these are only beneficial (e.g., Bacillus coagulans) or spoilage bacteria, and not related to foodborne illness. From a taste perspective, we’re extremely lucky that no thermophilic bacteria cause foodborne illness; otherwise, we’d have to cook foods to higher temperatures to kill them.
Salmonella gets most of the limelight in the media for a couple of reasons, though: it’s hardy—that is, able to survive in the environment for longer periods of time and at temperatures above what most other common-to-food bacteria can tolerate—and it’s surprisingly prevalent, affecting 1.4 million Americans a year on average.
Caliciviruses—a family of viruses, norovirus being the best known—are also getting more attention these days, and deservedly so; these are typically spread by a sick individual preparing food for others. If you’ve spent a night “praying to the porcelain god”—diarrhea, vomiting, chills, headache—you can probably thank salmonella or norovirus for the experience.
Now, pay attention, because this is important. Salmonella is killed at 136°F / 58°C only when held for a sufficient length of time. Seeing your thermometer register an even hotter temperature—say, 140°F / 60°C—does not guarantee that the food will be free of salmonella. Think of it like being in a hot desert: you can survive 136°F / 58°C heat for a while, but if you’re exposed to it for too long, eventually you will die. The same is true for bacteria like salmonella: given a short amount of time at a particular temperature, the bacteria might survive, but given a longer exposure, they will eventually die.
Salmonella actually lives in a temperature range of 35–117°F / 2–47°C according to the FDA’s “Bad Bug Book.” The 136°F / 58°C temperature is based on what the FDA Food Code gives as the lower bound for pasteurization.
Back to the desert analogy. Let’s say an average human can survive for four hours in 136°F / 58°C heat. Given 100 people in a desert, though, this doesn’t mean all 100 people will be alive at 3 hours, 59 minutes and all suddenly drop dead one minute later. The same is true for bacteria that might be hitching a ride on that chicken you’re about to cook: the proteins in the bacteria don’t all spontaneously denature at a specific temperature. It’s a probability thing: as the temperature goes up, the probability of the molecular structure of each kind of protein denaturing increases. There’s not an exact temperature at which this occurs, like there is when a solid melts into a liquid.
Note
When talking about reducing the number of bacteria in food, scientists use the term log10 reductions. A single log10 reduction is simply the reduction of the number of bacteria present by a factor of 10; a 7 log10 reduction is a 10,000,000-fold reduction. The USDA’s Food Safety and Inspection Service (FSIS) division is responsible for providing guidelines relating to the number of log reductions necessary to achieve an acceptable quantity of bacteria. Given that different kinds of meats have different properties—different amounts of fats, water, etc.—the number of log reductions necessary to reduce the bacterial count from a potential starting amount to an acceptable number differs. Hold time for sufficient pasteurization is also affected by variables such as how smooth the surface of the food is and its chemical composition (e.g., nitrite levels).
One important caveat about pasteurization: sometimes it’s not the bacteria themselves that are the issue, but the toxins they produce. While appropriate cooking might safely reduce the bacterial count, the toxins themselves, such as those produced by B. cereus, can be heat-stable. Refrigeration of meats is therefore critical to prevent the multiplication of bacteria in the meat tissue. Remember the simple food safety rule mentioned earlier: avoid holding foods at temperatures between 40°F / 4°C and 140°F / 60°C for more than two hours. This includes the amount of time it takes to bring the food from fridge temperature to a safe hot temperature! While it is true that the 40-to-140°F / 4-to-60°C rule for two hours is a vast simplification of the real multiplication rates of bacteria, it’s a simple rule accepted by the food industry, and rarely is there any need to skirt it.
At 140°F / 60°C, a hold time of 35 minutes is necessary for chicken with 12% fat to achieve a 7-log10 reduction. The time drops in leaner chickens; chicken meat with 1% fat requires 25.2 minutes at 140°F / 60°C. Longer hold times are okay; these times are minimum times. Chicken meats can be infected with salmonella throughout the tissue. While sick birds are supposed to be culled, it’s still possible for them to go unnoticed. (Data from http://www.fsis.usda.gov/OPPDE/rdad/FSISNotices/RTE_Poultry_Tables.pdf.)
“Why then,” I bet you’re thinking, “do ‘they’ say to cook chicken to a temperature of 165°F / 74°C?” “They” happen to be the fine folks at the CDC, and what they say specifically is:
All poultry should be cooked to reach a minimum internal temperature of 165°F [74°C].
Why 165°F / 74°C? One reason is that this is the temperature at which salmonella dies a quick death. From a “keeping it simple” perspective, seeing 165°F / 74°C on the thermometer is an easy guideline. Even if your thermometer is miscalibrated or you misprobe the meat and it’s only reached a temperature of 155°F / 68°C, the pasteurization time for chicken at this temperature is less than a minute, which you’re likely to exceed. The 165°F / 74°C guideline effectively removes the variable of time, making it an easier to follow (harder to screw up) rule.
Since none of the bacteria related to foodborne illness can survive, let alone reproduce, at moderate temperatures, holding food above 140°F / 60°C indefinitely is safe. This is why the soup at your local lunch counter can be kept hot all day long in a heat-controlled container and why hot buffets use steam baths to keep the foods warm. While you might be perplexed by the idea of storing foods hot, from a bacterial control perspective, it’s actually safer than storing them in the fridge: bacteria are unable to survive in the hot environment, while storing them in the fridge generally only slows their reproduction.
Note
The serving spoons, by the way, are supposed to stay in the food, so that they too stay above 140°F / 60°C. Otherwise, that mashed potato clinging to the serving spoon at room temperature will be a potential hangout spot for bacteria.
In the U.S., the FSIS and the FDA run testing programs to monitor the food supply. Both agencies have the ability to hold foods at processing plants, to request voluntary recalls, and to outright seize product through court order if it comes to that. Still, there’s a lot of food going through the system, and lapses in protocol happen (probably more than we want to know about). A lot of work is done in identifying hazard points in the food system (HACCP—Hazard Analysis & Critical Control Points), but still, errors happen. What’s a nervous food geek to do?
The most common vector for foodborne illness is surface contamination, either from contaminated water sprayed on vegetables during farming or from fecal contamination in meats during slaughter and processing. How does this affect you when cooking? Since it’s the surface of most products that becomes contaminated, it’s the surface that needs to be pasteurized. Pan searing a steak heats the outer portion well beyond any temperature that bacteria can survive. Likewise, steaming vegetables thoroughly heats their surface.
Note
When cooking vegetables in the microwave, use a container with the lid mostly closed and with a small amount of water inside: the microwave will boil the water, and the container will keep the steam in contact with the vegetables.
What about hamburgers? Well, they’re all outside, in the sense that surface contamination will have been ground throughout the meat. Industry calls things like steak whole-muscle intact meat, as opposed to ground meat. When looking at consumer cooking guidelines, the temperatures given are lower for whole-muscle intact than ground meats, presumably because the outside of a whole muscle cut will be well beyond pasteurized by the time the middle comes to temperature.
Note
For a little light reading, pull up the FDA’s 2009 Food Code (http://www.fda.gov/Food/FoodSafety/RetailFoodProtection/FoodCode/) and look at section 3-401.11: Raw Animal Foods.
When cooking a hamburger, the USDA says to heat the meat to 160°F / 71.1°C—high enough to kill any common bacteria but also high enough that both actin and myosin proteins will denature, leading to a drier burger. Since fats help mask dryness in meat, using ground beef that has more fat in it will lead to a juicer burger. Alternatively, if you have a way of cooking your burger to a lower temperature and then holding it at temperature long enough to pasteurize it, you could avoid denaturing the actin proteins while still pasteurizing the meat. Take a look at the section on sous vide cooking in Sous Vide Cooking of Chapter 7 for more on this.
Note that change in color is not an accurate indicator of doneness. Myoglobin, oxymyoglobin, and metmyoglobin can begin to turn grey starting around 140°F / 60°C, and they can also remain pink at 160°F / 71°C if the pH is at or about 6.0. Use a thermometer when cooking ground meats and poultry!
How to Prevent Foodborne Illness Caused by Parasites
Not long ago, I overheard the fishmonger at one of my local grocery stores (which shall remain nameless to protect the guilty) tell a customer that it was okay to use the salmon he was selling for making sushi. Given that the fish wasn’t labeled as “previously frozen” and that it was in direct contact with other fish in the case, there wasn’t any real guarantee that it was free from harmful parasites or bacteria, two of the biggest concerns that consumers need to manage for food safety. What’s a shopper to do in response to the disappearance of the true fishmonger?
For one, start by understanding where the risks actually are. Not all fish and meats share the same set of risks for foodborne pathogens. Salmonella, for example, tends to show up in land animals and improperly handled vegetables, while bacteria such as Vibrio vulnificus show up in fish that are exposed to the brackish waters of tidal estuaries, such as salmon. Deep-water fish, such as tuna, are of less concern. Because of these differences, you should consider the source of your ingredients when thinking about food safety, focusing on the issues that are present in the particular food at hand.
With uncooked and undercooked fish, one concern is parasites. Parasites are to fish as bugs are to veggies: really common (if you’ve eaten fish, you’ve eaten worms). But on the plus side, most parasites in seafood don’t infect humans. However, there are those that do, Anisakis simplex and tapeworms (cestodes) being the two parasites of general concern. A. simplex will give you abdominal pains, will possibly cause you to vomit and generally feel like crap, and will possibly take your doc a while to figure out. It’s not appendicitis, Crohn’s disease, nor a gastric ulcer, and with only around 10 cases diagnosed per year in the United States, chances are your doc won’t have encountered it before. On the plus side, humans are a dead-end host for A. simplex. The bacteria will die after about 10 days, at which point you’ll go back to feeling normal. (Unless you have an extreme infection, in which case, it’s off to surgery to remove ’em.) That leaves tapeworms as the major parasitic concern in fish.
For cooked dishes—internal temperature of 140°F / 60°C—there is little risk from these parasites directly. Cooking the fish also cooks the parasite, and while the thought of eating a worm might be unappetizing, if it’s dead there’s little to worry about other than the mental factor. (Just think of it as extra protein.)
Of course, raw and undercooked seafood is another matter entirely. Cod, halibut, salmon? Fish cooked rare or medium rare? Ceviche, sashimi, cold-smoked fish? All potential hosts for roundworm, tapeworms, and flukes. Fortunately, like most animals, few parasites can survive freezing.
Note
Some parasites do survive freezing. Trichomonas—parasitic microorganisms that infect vertebrates—can survive temperatures as cold as liquid nitrogen. Yikes!
For the FDA to consider raw or undercooked fish safe to eat, it must be frozen for a period of time to kill any parasites that might be present:
FDA 2005 Food Code, Section 3-402.11: “[B]efore service or sale in ready-to-eat form, raw, raw-marinated, partially cooked, or marinated-partially cooked fish shall be: (1) Frozen and stored at a temperature of –20°C (–4°F) or below for a minimum of 168 hours (7 days) in a freezer; [or] (2) Frozen at –35°C (–31°F) or below until solid and stored at –35°C (–31°F) or below for a minimum of 15 hours...”
The second concern with undercooked fish is bacteria. While freezing kills parasites, it does not kill bacteria; it just puts them “on ice.” Researchers store bacterial samples at –94°F / –70°C to preserve them for future study, so even super-chilling food does not destroy bacteria. Luckily, most bacteria in fish can be traced to surface contamination due to improper handling—that is, cross-contamination from surfaces previously exposed to contaminated items.
Note
Don’t put cooked fish or meat on the same plate as the raw food! In addition to being potentially dangerous, that’s just gross.
If your grocery store sells both raw and “sashimi-grade” fish, the difference between the two will be in the handling and care related to the chances of surface contamination, and in most cases the sashimi-grade fish should have been previously frozen. The FDA doesn’t actually define what “sashimi grade” or “sushi grade” means, but it does explicitly state that fish not intended to be completely cooked before serving must be frozen before being served.
If you don’t have access to a good fish market or find the frozen fish available at your local grocery store unappealing, and you plan on serving undercooked fish, you can kill any parasites present in the fish by freezing: check that your freezer is at least as cold as –4°F / –20°C, and follow the FDA rule of keeping the fish frozen for a week. If you happen to have a supply of liquid nitrogen around—you know, just by chance—you can also flash-freeze the fish, which should result in better texture and cut the hold time down to less than a day.
Luckily for oyster lovers, the FDA excludes
molluscan shellfish, as well as some types of tuna and some
farm-raised fish (those that are fed only food pellets that wouldn’t
contain live parasites) from the freezing requirement.
Key Temperatures in Cooking
Most discussions of cooking are structured around the different heat transfer methods listed at the beginning of this chapter. Instead of looking at sources of heat, the rest of this chapter is going to take a different approach and talk about what reactions happen when each of the critical temperatures in the following table is reached, briefly touching on cooking techniques that relate to each temperature as they come up.
Temperature | What happens |
104°F / 40°C and 122°F / 50°C | Proteins in fish and meat begin to denature |
144°F / 62°C | Eggs begin to set |
154°F / 68°C | Collagen denatures (Bovine Type I) |
158°F / 70°C | Vegetable starches gelatinize |
310°F / 154°C | Maillard reactions become noticeable |
356°F / 180°C | Sugar (sucrose) begins to caramelize visibly |
104°F / 40°C and 122°F / 50°C: Proteins in Fish and Meat Begin to Denature
Chances are, you haven’t given much thought to the chemical reactions that happen to a piece of meat when the animal supplying it is slaughtered. The primary change is, to put it bluntly, that the animal is dead, meaning the circulatory system is no longer supplying the muscle tissue with glycogen from the liver or oxygen-carrying blood. Without oxygen, the cells in the muscle die, and preexisting glycogen in the muscle tissue dissipates, causing the thick and thin myofilaments in the muscle to fire off and bind together (resulting in the state called rigor mortis).
Somewhere around 8 to 24 hours later, the glycogen supply is exhausted and enzymes naturally present in the meat begin to break down the bonds created during rigor mortis (postmortem proteolysis). Butchering before this process has run its course will affect the texture of the meat. Sensory panels have found that chicken breasts cut off the carcass before rigor mortis was over have a tougher texture than meat left on the bone longer. And since time is money, much mass-produced meat is slaughtered and then butchered straightaway. (I knew there was a reason why roasted whole birds taste better!)
Proteins in meat can be divided into three general categories: myofibrillar proteins (found in muscle tissue, these enable muscles to contract), stromal proteins (connective tissue, including tendons, that provide structure), and sarcoplasmic proteins (e.g., blood). We’ll talk about myofibrillar proteins here and save the stromal proteins for the section on collagen later in the chapter. (We’re going to ignore sarcoplasmic proteins altogether, because understanding them doesn’t help in cooking many dishes, blood-thickened soups aside.)
Muscle tissue is primarily composed of only a few types of proteins, with myosin and actin being the two most important types in cooking. About two-thirds of the proteins in mammals are myofibrillar proteins. The amount of actin and myosin differs by animal type and region. Fish, for example, are made up of roughly twice as much of these proteins as mammals.
Lean meat is mostly water (65–80%), protein (16–22%), and fat (1.5–13%), with sugars such as glycogen (0.5–1.3%) and minerals (1%) contributing only a minor amount of the mass. When it comes to cooking a piece of fish or meat, the key to success is to understand how to manipulate the proteins and fats. Although fats can be a significant portion of the mass, they are relatively easy to manage, because they don’t provide toughness. This leaves proteins as the key variable in cooking meats.
Of the proteins present in meat, myosin and actin are the most important from a culinary texture perspective. If you take only one thing away from this section, let it be this: denatured myosin = yummy; denatured actin = yucky. Dry, overcooked meats aren’t tough because of lack of water inside the meat; they’re tough because on a microscopic level, the actin proteins have denatured and squeezed out liquid in the muscle fibers. Myosin in fish begins to noticeably denature at temperatures as low as 104°F / 40°C; actin denatures at around 140°F / 60°C. In land animals, which have to survive warmer environments and heat waves, myosin denatures in the range of 122–140°F / 50–60°C (depending on exposure time, pH, etc.) while actin denatures at around 150–163°F / 66–73°C.
Food scientists have determined through empirical research (“total chewing work” and “total texture preference” being my favorite terms) that the optimal texture of cooked meats occurs when they are cooked to 140–153°F / 60–67°C, the range in which myosin and collagen will have denatured but actin will remain in its native form. In this temperature range, red meat has a pinkish color and the juices run dark red.
The texture of some cuts of meat can be improved by tenderizing. Marinades and brines chemically tenderize the flesh, either enzymatically (examples include bromelain, an enzyme found in pineapple, and zingibain, found in fresh ginger) or as a solvent (some proteins are soluble in salt solutions). Dry aging steaks works by giving enzymes naturally present in the meat time to break down the structure of collagen and muscle fibers. Dry aging will affect texture for at least the first seven days. Dry aging also changes the flavor of the meat: less aged beef tastes more metallic, more aged tastes gamier. Which is “better” is a matter of personal taste preference. (Perhaps some of us are physiologically more sensitive to metallic tastes.) Retail cuts are typically 5 to 7 days old, but some restaurants use meat aged 14 to 21 days.
Then there are the mechanical methods for
“tenderizing,” which aren’t actually so much tenderizing as they are
masking toughness: for example, slicing muscle fibers against the
grain thinly, as is done with beef carpaccio and London broil, or
literally grinding the meat, as is done for hamburger meat. (Some
industrial meat processors “tenderize” meat by microscopically slicing
it using very thin needles, a method called jacquarding.) Applying
heat to meats “tenderizes” them by physically altering the proteins on
the microscopic scale: as the proteins denature, they loosen up and
uncurl. In addition to denaturing, upon uncurling, newly exposed
regions of one protein can come into contact with regions of another
protein and form a bond, allowing them to link to each other. This
process is called coagulation, and while it
typically occurs in cooking that involves protein denaturation, it is
a separate phenomenon.
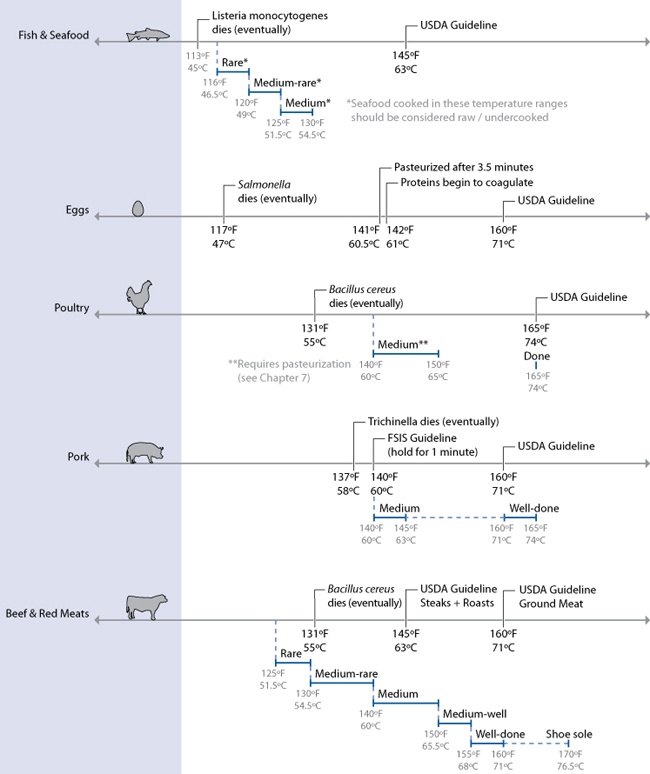
Temperatures required for various levels of doneness. Note that seafood cooked very rare or medium rare and chicken cooked medium must be held for a sufficiently long period of time at the stated temperature in order to be properly pasteurized. See the section on Sous Vide Cooking in Chapter 7 for time and temperature charts.
144°F / 62°C: Eggs Begin to Set
The lore of eggs is perhaps greater than that of any other food item, and more than one chef has gone on record judging others based on their ability—or inability—to cook an egg. Eggs are the wonder food of the kitchen—they have a light part, a dark part, and bind the culinary world together. Used in both savory and sweet foods, they act as binders holding together meatloaf and stuffing; as rising agents in soufflés, certain cakes, and cookies like meringues; and as emulsifiers in sauces like mayonnaise and hollandaise. Eggs provide structure to custards and body to ice creams. And all of this so far doesn’t even touch on their flavor or the simple joys of a perfectly cooked farm egg. Simply put, I cannot think of another ingredient whose absence would bring my cooking to a halt faster than the simple egg.
Egg whites are composed of dozens of different types of proteins, and each type of protein begins to denature at a different temperature. In their natural “native” state, you can think of the proteins as curled-up little balls. They take this shape because portions of the molecular structure are hydrophobic—the molecular arrangement of the atoms making up the protein is such that regions of the protein are electromagnetically repulsed by the polar charge of water.
Because of this aversion to water, the protein structure folds up on itself. As kinetic energy is added to the system—in the form of heat or mechanical energy (e.g., whipping egg whites)—the structure starts to unfold as kinetic energy overtakes potential energy. The unfolded proteins then get tangled together, “snagging” around other denatured proteins and coagulating to form a linked structure. This is why a raw egg white is liquid, but once cooked becomes solid. (Well, technically, raw egg white is a gel that coagulates into a solid-like substance when heated. We’ll get to gels in Chapter 6.)
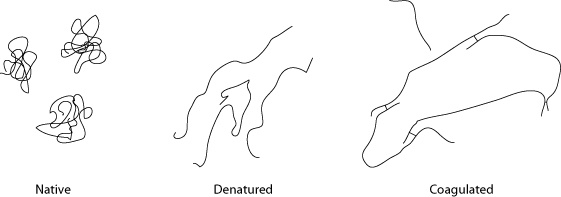
Hydrophobic proteins in their native state (left) remain curled up to avoid interacting with the surrounding liquid. Under heat, they denature (center) and uncurl as the kinetic energy exceeds the weaker level of energy generated by water molecules and regions of the proteins that repel each other. Once denatured and opened up, the hydrophobic parts of the protein that were previously unexposed can interact and bond with other proteins.
The most heat-sensitive protein is ovotransferrin, which begins to denature at around 144°F / 62°C. Another protein, ovalbumin, denatures at around 176°F / 80°C. These two proteins also are the most common in egg whites: ovotransferrin accounts for 12% of the proteins in an egg white and ovalbumin 54%. This explains the difference between soft-boiled and hard-boiled (“hard-cooked”) eggs. Get that egg up to about 176°F / 80°C for sufficient time, and voilà, the white is hard cooked; below that temperature, however, the ovalbumin proteins remain curled up, leaving the majority of the egg white in its “liquid” state.
Note
Most of the proteins in egg yolks set at between 149°F / 65°C and 158°F / 70°C, although some set at lower temperatures.
Proteins in foods such as eggs don’t denature
instantaneously once they reach denaturation temperature. This is an
important point. Some cooking newbies have the mental model that
cooking an egg or a piece of meat is something like melting an ice
cube: all ice below a certain temperature, ice and water at the
freezing/melting point, and all water above that temperature. From a
practical perspective in the kitchen, it’s not an entirely incorrect
picture, because heat pours into the foods so quickly that the subtle
differences between a few degrees aren’t obvious. But as heat is
transferred into the food more slowly, the subtleties of these
chemical reactions become more noticeable. And unlike melting an ice
cube, where increasing the heat transfer by a factor of two causes the
ice to melt in half the time, cooking foods do not respond to
additional energy in a linear fashion.
You might find it easiest to think of the different proteins in foods as having particular temperatures at which they denature, and try to shoot for a target temperature just above that of the proteins you do want denatured. Just remember: there’s more to a piece of meat or egg than one type of protein or connective tissue, and the different proteins have different temperature points at which they’re likely to denature.
Here are some examples of cooking eggs that show how to take advantage of the thermal properties of different portions of the egg.
Hard-Cooked Eggs, Shock and Awe Method
There’s a silent war of PC-versus-Mac proportions going on over the ideal way to make hard-cooked eggs. Should you start in cold water and bring the water up to a boil with the eggs in them, or should you drop the eggs into already boiling water? The cold-start approach yields eggs that taste better, while the boiling-water approach yields eggs that are easier to peel. But can you have both?
Thinking about the thermal gradient from shell to center of egg, it would make sense that cooking an egg starting in cold water would result in a more uniform doneness. The delta between the center and outer temperatures will be smaller, meaning that the outer portion won’t be as overcooked once the center is set compared to the boiling-water method.
The conjecture for ease of peeling in the boiling water approach is that the hot water “shocks” the outer portion of the egg. Into industrial-grade cooking? Steam ’em at 7.5 PSI over atmospheric pressure and quick-release the pressure at the end of cooking to crack the shell. (Hmm, I wonder if one could do this in a pressure cooker...) But what about the rest of us? What if we shock the outside, and then cook in cold water?
Try it. Place your eggs into rapidly boiling water. After 30 seconds, transfer the eggs to a second pot containing cold tap water, bring to a boil, and then simmer. The second-stage cooking time will take about two minutes less than the normal cold-start approach. Cook for 8 to 12 minutes, depending upon how well cooked you like your eggs.
The 30-Minute Scrambled Egg
This method involves ultra-low heat, continuous stirring, and a vigilant eye. I wouldn’t suggest this as an everyday recipe, because it takes a while to make, but after however many years of eating eggs, it’s nice to have them cooked a new way. Cooking the eggs over very low heat while continuously stirring breaks up the curds and allows for cooking the eggs to a point where they’re just cooked, giving them a flavor that can be described as cheese or cream-like. It’s really amazing, and while the thought of “cheese or cream-like” eggs might not have you racing off to the kitchen, it’s really worth a try!
In a bowl, crack two or three eggs and whisk thoroughly to combine the whites and yolks. Don’t add any salt or other seasonings; do this with just eggs. Transfer to a nonstick pan on a burner set to heat as low as possible.
Stir continuously with a silicone spatula, doing a “random walk” so that your spatula hits all parts of the pan. And low heat means really low heat: there’s no need for the pan to exceed 160°F / 71°C, because enough of the proteins in both the yolks and whites denature below that temperature and the proteins will weep some of their water as they get hotter. If your heat source is too hot, pull the pan off the stovetop for a minute to keep it from overheating. If you see any curds (lumps of scrambled eggs) forming, your pan is getting too hot.
Continue stirring until the eggs have set to a custard-like consistency. When I timed myself, this took about 20 minutes, but you might reach this point in as few as 15 minutes or upward of half an hour.
Oven-Poached Eggs
Here’s a simple way to cook eggs for a brunch or appetizer. In an individually sized oven-safe bowl (ideally, one that you can serve in), add:
Breakfast version | Dinner version |
1 cup (30g) fresh chopped spinach | ½ cup (100g) crushed tomatoes |
3 tablespoons (20g) grated mozzarella cheese | ¼ cup (50g) black beans (canned are easiest) |
3 tablespoons (40g) heavy cream | ½ cup (50g) grated mozzarella cheese |
4 teaspoons (20g) butter |
Create a “well” in the center of the ingredients by pushing the food into a ring around the edges of the bowl. Crack two eggs into the well, add a pinch of salt and some fresh ground pepper, cover with aluminum foil, and bake in a preheated oven set to 350°F / 180°C until the egg is set, about 25 minutes. (You can use a probe thermometer set to beep at 140°F / 60°C.) Try adding some crushed red pepper flakes to the breakfast version or sriracha sauce to the dinner version.
Pasteurized Eggs
While salmonella is quite rare in uncooked eggs, with estimates being somewhere around 1 in 10,000 to 20,000 eggs carrying the bacteria, it does occur in the laying hen populations of North America. If you’re cracking a few dozen eggs into a bowl for an omelet brunch at your local hacker house every week, let’s just say that odds are you’ll eventually crack a bad egg. Luckily, this isn’t a problem if those eggs are properly cooked and cross-contamination is avoided.
The real risk for salmonella in eggs is in dishes that use undercooked eggs that are then served to at-risk populations (e.g., infants, pregnant women, elderly or immunocompromised people). If you’re making a dish that contains raw or undercooked eggs—Caesar salad, homemade eggnog, mayonnaise, raw cookie dough—and want to serve that dish somewhere where there might be at-risk individuals, you can pasteurize the eggs (assuming your local store doesn’t happen to carry pasteurized eggs, but most don’t). Pasteurized eggs do taste a little different, and the whites take longer to whip into a foam, so don’t expect them to be identical to their raw counterparts.
Since salmonella begins to die at a noticeable rate around 136°F / 58°C and the proteins in eggs don’t begin to denature until above 141°F / 61°C, you can pasteurize eggs to reduce the quantity of salmonella, should it be present, to an acceptable level by holding the egg at a temperature between these two points. The FDA requires a 10,000-fold reduction (5 log10 in food safety lingo), which can be achieved by holding the egg at 141°F / 61°C for 3.5 minutes (according to Margaret McWilliams’s Foods: Experimental Perspectives, Fifth Edition, from Pearson Publishing). Most consumers won’t have the necessary hardware to do this at home, but if you do have a sous vide setup, as described in Chapter 7, you’re golden.
The 60-Minute Slow-Cooked Egg
Going back to our earlier discussion of time and temperature, when food is left in an environment long enough, its temperature will come to match that of its environment. Therefore, if we immerse an egg in water held at 145°F / 62.7°C, it follows that the proteins in the white and the yolk that denature at or below that temperature will denature and coagulate, and those that denature above that temperature will remain unaltered.
The added benefit of this method is that the egg cannot overcook. “Cooking” is effectively the occurrence of chemical reactions in the food at different temperature points, and holding the egg at 145°F / 62.7°C will not trigger any reactions that don’t occur until higher temperatures are reached. This is the fundamental concept of sous vide cooking. We’ll cover the details of sous vide in Chapter 7, so you may want to take a peek at that chapter now or make a mental note to come back to this section when you get there. For a sous vide–style cooked egg, immerse an egg in water that is maintained at 145°F / 62.7°C for one hour. As you’ll see, sous vide cooking has some incredible properties that greatly simplify the time and temperature rule.
Note
Your average, run-of-the-mill (or is that run-of-the-yard?) chicken laid only 84 eggs per year a century ago. By the turn of the millennium, improvements in breeding and feed had pushed this number up to 292 eggs per year—almost 3.5 times more. And, no, science has not yet figured out which came first.
154°F / 68°C: Collagen (Type I) Denatures
An animal’s connective tissues provide structure and support for the muscles and organs in its body. You can think of most connective tissues—loose fascia and ligaments between muscles as well as other structures such as tendons and bones—as a bit like steel reinforcement: they don’t actively contract like muscle tissue, but they provide structure against which muscles can pull and contract.
The most common type of protein in connective tissue is collagen, and while there are several types of collagen in animals, from a culinary perspective, the main chemical difference between the different types of collagen is the temperature at which they denature. In cooking, collagen shows up in two different ways: either as discrete chunks (e.g., tendons, silverskin) outside of the muscle, or as a network that runs through the muscle. Regardless of its location, collagen is tough (it provides structure, after all) and becomes palatable only given sufficient time at sufficiently high temperatures.
It’s easy to deal with collagen that shows up as discrete pieces: get rid of it by cutting it off. For cuts of meats that have a thin layer of connective tissue on them (called silverskin, presumably because of its somewhat iridescent appearance), cut off as much as possible and discard it. Beef tenderloin cuts commonly have a side with this layer; trim off as much as possible before cooking.
Chicken breasts also have a small but noticeable tendon connected to the chicken tenderloin. Uncooked, it’s a pearlescent white ribbon. After cooking, it turns into that small white rubber-band-like thing that you can chew on endlessly yet never get any satisfaction from. Generally, this type of collagen is easy to spot, and if you miss it, it’s easy to notice while eating and can be left on the plate.
However, for the other kind of collagen found in some cuts of meat—collagen that forms a 3D network through the muscle tissue—the only way to remove it is to convert it to gelatin via long, slow cooking methods. Unlike muscle proteins—which in cooking are either in a native (i.e., as they are in the animal), denatured, or hydrolyzed state—collagen, once hydrolyzed, can enter a coagulated (gelled) state. This property opens up an entirely new world of possibilities, because gelatin gives meats a lubricious, tender quality and provides a lip-smacking goodness.
In its native form, collagen is like a rope: it’s a linear molecule composed of three different strands that are twisted together. The three strands are held together by weak secondary bonds (but there are a lot of them!) and stabilized by a small number of crosslinks, which are stronger covalent bonds.
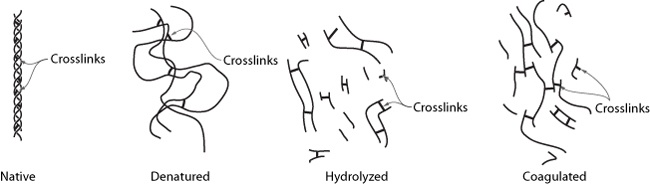
Collagen in its native form is a triple helix, held together in its helical structure by secondary bonds (left) and stabilized by crosslinks. Under heat, the secondary bonds break and the protein becomes denatured, but the crosslinks between the strands continue to hold the structure together (second from left). Given sufficient heat and time, the strands in the triple helix themselves break down via hydrolysis (third from left) and, upon cooling, convert to a loose network of molecules (right) that retains water (a gel).
Note
Covalent bonds are bonds where the electrons from an atom in one location are shared with another atom.
In addition to being crosslinked, the strands also form a helical structure because of secondary bonds between different regions of the same molecules. You can think of it something like a braided rope, where each strand wraps around the other two strands. It has a “curl” to it because the internal structure finds its optimal resting place in that shape.
Under the right conditions—usually, exposure to heat or the right kinds of acids—the native form of collagen denatures, losing its linear structure and untwisting into a random mess. With the addition of sufficient heat, the molecules in the structure will vibrate enough to overcome the electromagnetic energy that caused the structure to twist up in the first place, leading it to lose its helical structure and denature.
Acids can also denature the collagen protein: their chemical properties provide the necessary electromagnetic pull to disrupt the secondary bonds of the helical structure. It’s only the twisting that goes away during denaturing in collagen; the crosslinks remain in place and the strands remain intact. In this form, collagen is like rubber—it actually is a rubber from a material science point of view—and for this reason, you’ll find its texture, well, rubbery.
Given even more heat or acid, though, the collagen structure undergoes another transformation: the strands themselves get chopped up and lose their backbone, and at this point the collagen has no real large-scale structure left. This reaction is called hydrolysis: thermal hydrolysis in the case of heat, acid hydrolysis in the case of, you guessed it, acid. (Think ceviche. See the section on Acids and Bases in Chapter 6 for more.)
It’s possible to break up the collagen chemically, too: lysosomal enzymes will attack the structure and “break the covalent bonds” in chem-speak, but this isn’t so useful to know in the kitchen.
Note
For fun, try marinating a chunk of meat in papaya, which contains an enzyme, papain, that acts as a meat tenderizer by hydrolyzing collagen.
One piece of information that is critical to understand in the kitchen, however, is that hydrolysis takes time. The structure has to literally untwist and break up, and due to the amount of energy needed to break the bonds and the stochastic processes involved, this reaction takes longer than simply denaturing the protein.
Hydrolyzing collagen not only breaks down the rubbery texture of the denatured structure, but also converts a portion of it to gelatin. When the collagen hydrolyzes, it breaks into variously sized pieces, the smaller of which are able to dissolve into the surrounding liquid, creating gelatin. It’s this gelatin that gives dishes such as braised ox tail, slow-cooked short ribs, and duck confit their distinctive mouthfeel.
Since these dishes rely on gelatin for providing that wonderful texture, they need to be made with high-collagen cuts of meat. Trying to make a beef stew with lean cuts will result in tough, dry meat. The actin proteins will denature (recall that this occurs at temperatures of 150–163°F / 66–73°C), but the gelatin won’t be present in the muscle tissue to mask the dryness and toughness brought about by the denatured actin. Don’t try to “upgrade” your beef stew with a more expensive cut of meat; it won’t work!
“Great,” you might be thinking, “but how does any of this tell me whether I need to slow-cook a piece of meat?” Think about the piece of meat (or fish or poultry) that you’re working with and consider what part of the animal it comes from. For a land-based animal, those regions of the animal that bear weight generally have higher levels of collagen. This should make sense: because the weight-bearing portions have a higher load, they need more structure, so they’ll have more connective tissue. This isn’t a perfect rule of thumb, though, and cuts of meat generally have more than one muscle group in them.
For animals like fish, which don’t have to support their weight on land, the collagen levels are much lower. Squid and octopus are notable exceptions to this weight-bearing rule, because their collagen provides the equivalent support that bone structures do for fish.
Note
Older animals have higher levels of collagen. As animals age, the collagen structure has more time to form additional crosslinks between the strands in the collagen helix, resulting in increased toughness. This is why older chickens, for example, are traditionally cooked in long, slow roasts. (The French go so far as to use different words for old versus young chickens: poule instead of poulet.) Most commercial meat, however, is young at time of slaughter, so the age of the animal is no longer an important factor.
The other easy rule of thumb for collagen levels is to look at the relative price of the meat: because high-collagen cuts require more work to cook and come out with a generally drier texture, people tend to favor other cuts, so the high-collagen cuts are cheaper.
158°F / 70°C: Vegetable Starches Break Down
Whereas meat is predominately proteins and fats, plants are composed primarily of carbohydrates such as cellulose, starch, and pectin. Unlike proteins in meat, which are extremely sensitive to heat and can quickly turn into shoe leather if cooked too hot, carbohydrates in plants are generally more forgiving when exposed to higher temperatures. (This is probably why we have meat thermometers but not vegetable thermometers.)
Cooking starchy vegetables such as potatoes causes the starches to gelatinize (i.e., swell up and become thicker). In their raw form, starches exist as semicrystalline structures that your body can only partially digest. Cooking causes them to melt, absorb water, swell, and convert to a form that can be more easily broken down by your digestive system.
As with most other reactions in cooking, the point at which starch granules gelatinize depends on more than just the single variable of temperature. The type of starch, the length of time at temperature, the amount of moisture in the environment, and processing conditions all impact the point at which any particular starch granule swells up and gelatinizes. See the section Making gels: Starches in Chapter 6 for more about starches and gelatinization.
Leafy green vegetables also undergo changes when cooked. Most noticeably, they lose their green color as the membranes around the chloroplasts in the cells rupture. This same rupturing and damage to the cell structure is what improves the texture of tougher greens such as Swiss chard and kale.
For starchy plants (think potatoes), cook them so that they reach the temperature at which they gelatinize, typically in the range of 180–190°F / 92–99°C. For green leafy plants, sauté the leaves above 140°F / 60°C to break down the plant cell structure.
310°F / 154°C: Maillard Reactions Become Noticeable
The Maillard reaction turns foods brown and generates mostly pleasant volatile aromatic compounds. You can thank Maillard reactions for the nice golden-brown color and rich aromas of a Thanksgiving turkey, Fourth of July hamburger, and Sunday brunch bacon. If you’re still not able to conjure up the tastes brought about by Maillard reactions, take two slices of white bread and toast them—one until just before it begins to turn brown, the second until it has a golden-brown color—and taste the difference.
The nutty, toasted, complex flavors generated by the Maillard reaction are created by the hundreds of compounds formed when amino acids and certain types of sugars combine and then break down. Named after the French chemist Louis Camille Maillard, who first described it in the 1910s, the Maillard reaction is specifically a reaction between amino acids (from proteins) and reducing sugars, which are sugars that form aldehydes or ketone-based organic compounds in an alkaline solution (which allows them to react with the amines). Glucose, the primary sugar in muscle tissue, is a reducing sugar; sucrose (common table sugar) is not.
Maillard reactions aren’t solely dependent on temperature. Besides temperature, there are a number of other variables that affect the reaction rate. More alkaline foods undergo Maillard reactions more easily. Egg whites, for example, can undergo Maillard reactions at the lower temperatures and higher pressure found in a pressure cooker. The amount of water and the types and availability of reactants in the food also determine the rate at which Maillard reactions will occur. It’s even possible for Maillard reactions to happen at room temperature, given sufficient time and reagents: self-tanning products work via the same chemical reaction!
All things considered, though, in culinary applications—cooking at moderately hot temperatures for short periods of time—the 310°F / 154°C temperature given here serves as a good marker of when Maillard reactions begin to occur at a noticeable rate, whether you’re looking through your oven door or sautéing on the stovetop.
356°F / 180°C: Sugar Begins to Caramelize Visibly
Unlike the Maillard reaction, which requires the presence of both amino acids and sugars and has a number of interdependent variables influencing the particular temperature of reaction, caramelization (the decomposition via dehydration of sugar molecules such as sucrose) is relatively simple, at least by comparison. Pure sucrose melts at 367°F / 186°C; decomposition begins at lower temperatures (somewhere in the range of 320–340°F / 160–170°C) and continues up until around 390°F / 199°C. (Melting is not the same thing as decomposition—sucrose has a distinct melting point, which can be used as a clever way of calibrating your oven. For more, see The Two Things You Should Do to Your Oven RIGHT NOW of Chapter 2.)
Like the Maillard reaction, caramelization results in hundreds of compounds being generated as a sugar decomposes, and these new compounds result in both browning and the generation of enjoyable aromas in foods such as baked goods, coffee, and roasted nuts. For some foods, these aromas, as wonderful as they might be, can overpower or interfere with the flavors brought by the ingredients, such as in a light gingersnap cookie or a brownie. For this reason, some baked goods are cooked at 350°F / 177°C or even 325°F / 163°C so that they don’t see much caramelization, while other foods are cooked at 375°F / 191°C or higher to facilitate it.
When cooking, ask yourself if what you are cooking is something that you want to have caramelize, and if so, set your oven to at least 375°F / 191°C. If you’re finding that your food isn’t coming out browned, it’s possible that your oven is running too cold. If items that shouldn’t be turning brown are coming out overdone, your oven is probably too hot.
Fructose, a simpler form of sugar found in fruit and honey, caramelizes at a lower temperature than sucrose, starting around 230°F / 110°C. If you have other constraints on baking temperature (say, water content in the dough prevents it from reaching a higher temperature), you can add honey to the recipe. This will result in a browner product, because the largest chemical component in honey is fructose (~40% by weight; glucose comes in second at ~30%).
Get Cooking for Geeks now with the O’Reilly learning platform.
O’Reilly members experience books, live events, courses curated by job role, and more from O’Reilly and nearly 200 top publishers.